3 Ecosystem condition, drivers of change and impacts in Norway’s ocean areas – status and trends
Climate change and rising ocean temperatures are having increasingly marked impacts on ecosystem condition in Norwegian waters. Anthropogenic climate change has already resulted in changes in the ocean climate in all three management plan areas. These are reflected for example in higher water temperatures, shrinking sea ice cover in the Barents Sea and coastal water darkening (increased light attenuation) in the North Sea and Skagerrak. Rising CO2 emissions are also making the oceans more acidic. These changes have in turn led to changes in ecosystem condition (see Box 3.1).
The impacts of climate change are becoming apparent in the Barents Sea–Lofoten area in the form of higher sea temperatures and a decline in sea ice. Climate change has had negative impacts on various populations of Arctic seabirds, marine mammals and fish. These impacts have to some extent been reversed in recent years as a result of a certain decline in sea temperature. The evidence does not indicate that there are impacts on the ecosystem as a whole, but this conclusion is very uncertain because only short time series are available for many ecological groups. There appears to have been some reduction in the impacts of fisheries in the Barents Sea in recent years. The large fish stocks play a key role in ecosystem dynamics and are also commercially important. The major Barents Sea stocks are generally in good condition, but poor recruitment is resulting in a decline in condition.
Temperatures are also rising in the Norwegian Sea as a result of climate change. Ecological condition has only been assessed for the pelagic ecosystem in this management plan area. Changes observed here include a decline in the stocks of mackerel and Norwegian spring-spawning herring. Catches have been far higher than the recommended TACs (total allowable catches) after the international system for sharing the TACs collapsed in 2013. There has also been a considerable decline in seabird populations.
Climate change has had a substantial impact on ecological condition in the North Sea and Skagerrak, particularly in the form of higher sea temperatures. The changing abiotic conditions together with other drivers of change, including the fisheries, are having major impacts on the rest of the ecosystem. In the North Sea and Skagerrak, changes have been observed in key groups of zooplankton, fish stocks, seabirds and benthic communities.
The situation for Norwegian seabirds is critical. It is estimated that the number of Norwegian seabirds has declined by more than 80 % in the period 1970–2020. Red-listed species make up 63 % of the species typically categorised as seabirds. More and more populations are showing a dramatic fall in numbers. Populations of species that breed along the coast of the North Sea and Skagerrak are in particularly poor condition. For most seabird species, the decline is a result of food shortages combined with climate change.
The status of a number of threatened species and habitat types has been deteriorating in recent years. Some improvements have also been observed, but for a considerably smaller number of species and habitats. Alien species can have major impacts on marine ecosystems, and in Norwegian waters are largely found along the coast. The number of alien species registered is highest in the south of Norway, and has risen in the past ten years.
Long-range transport of hazardous substances with air and ocean currents is the main source of pollution in the management plan areas. These inputs have been decreasing since measurement series started from the 1990s onwards, but this trend has levelled off to some extent in recent years. Hazardous substances are found in all Norwegian waters, and their bioaccumulation poses a health risk for seabirds and marine mammals at the highest trophic levels of food chains. However, seafood from Norwegian waters is safe to eat, since concentrations of contaminants are generally below the maximum permitted levels for food safety in edible parts of most species. Measures such as prohibiting catches or advising against consumption are introduced if levels of contaminants are found to be above the maximum permitted concentrations.
Coastal waters inside the baseline are included in the area considered in the scientific basis for the management plans (see Chapter 2). Ecosystems in coastal waters are under considerable pressure from a range of activities, including construction and other permanent occupation of areas, fisheries, dredging, aquaculture, land reclamation, shipping, recreation, fishing tourism and petroleum-related activities, and processes including discharges and runoff. Activities in coastal waters may have impacts on the marine environment further out to sea, for example through harvesting of species that move between coastal waters and the open sea (e.g. from spawning areas in fjords), or through degradation of spawning and nursery areas for such species in coastal waters.
Inputs of nutrients (phosphorus and nitrogen) and copper to coastal waters have risen considerably, mainly as a result of increasing discharges from coastal aquaculture facilities. Continual movement in the water masses results in water exchange between fjords, coastal waters and the open ocean. However, it is unclear what proportion of these pollutants is transported from coastal waters into the open sea. Eutrophication is a problem in coastal waters in some areas, including the Oslofjord, but not in the open ocean.
Large quantities of plastic pollution and marine litter are still being registered along the coast, on beaches, during seabed mapping, in trawls and in the stomachs of seabirds and other animals.
Up to 2100, it is expected that warming will intensify in Norwegian waters and that marine heatwaves will become more frequent, resulting in growing pressure on marine ecosystems. The seawater will become more acidic. There will also be a decline in oxygen levels, but this is not expected to be a limiting factor for marine organisms. Many species and habitats will suffer negative impacts and will either shift their distribution or be lost.
Textbox 3.1 Assessing ecosystem condition
A scientific panel for each of the management plan areas has assessed ecosystem condition in the three areas as part of the scientific basis for this white paper. This provides an overall assessment of the extent to which the ecosystems in an area are affected by human activity. The system has been incorporated into the work of the Advisory Group on Monitoring and supplements the information provided by the set of indicators used in the monitoring system for the marine environment.
In the assessments of ecosystem condition, a deviation from good condition is defined as meaning that the ecosystem has changed from the reference condition, which is called ‘intact nature’. ‘Intact nature’ refers to ecosystems that show little impact of modern industrial activities, including anthropogenic climate change. This means that in practice, what has been assessed is the extent to which an ecosystem is influenced by anthropogenic drivers. Ecosystem condition has been assessed for each of the three management plan areas using seven ecosystem characteristics that together cover the main features of structures and processes in the ecosystems. The seven characteristics are primary productivity; biomass distribution among trophic levels; functional groups within trophic levels; functionally important species and biophysical structures; landscape-ecological patterns: biological diversity; and abiotic factors. After an overall assessment of condition for each ecosystem characteristic, the condition of the ecosystem as a whole is assessed. An ecosystem that is in good condition does not show any substantial deviation from the reference condition.
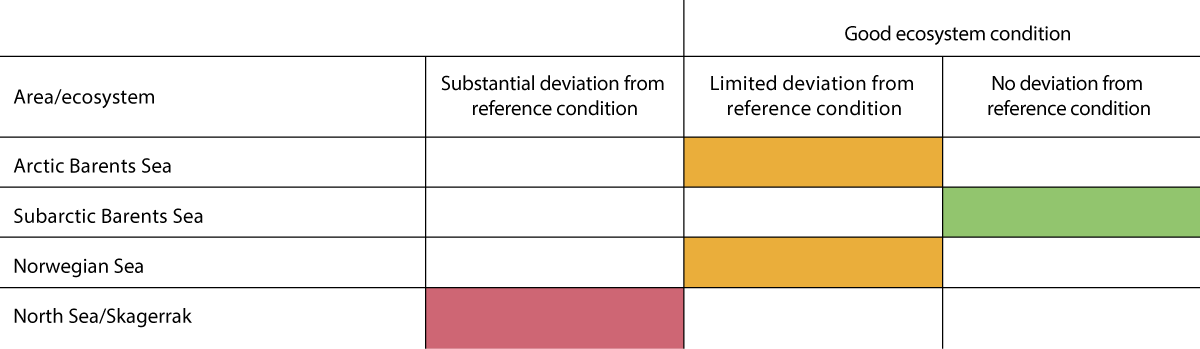
Figure 3.1 Overall assessment of ecosystem condition in the three management plan areas.
Source: Norwegian Environment Agency/miljøstatus.no
Assessment of cumulative impacts
As part of the scientific basis for this white paper, a report has been published describing risk assessments of the cumulative impacts of pressures in each of the particularly valuable and vulnerable areas in the three management plan areas (North Sea–Skagerrak, Norwegian Sea and Barents Sea–Lofoten), based on information on activity in various sectors from a representative period (2017–2019). This is a first trial of risk-based assessment of cumulative impacts, using a preliminary version of the methodology. So far, the risk-based assessments indicate wide variation in the risks associated with cumulative impacts in the various particularly valuable and vulnerable areas identified in Norwegian waters. The highest risk of adverse impacts is in the areas closest to the coast, where there is activity in the largest number of sectors and the degree of exposure to various pressures is high.
3.1 Ecosystem condition, drivers of change and impacts in the Barents Sea–Lofoten management plan area
Climate change is an important driver in the Barents Sea–Lofoten area, and has probably already altered both ecosystem structure and functioning, particularly in the Arctic part of the management plan area. The fisheries are another important driver in this area, in addition to anthropogenic climate change, although some of the impacts of the fisheries appear to have diminished in recent years. Over the past 50 years, there has been a severe decline in seabird populations in all three management plan areas. In the Barents Sea–Lofoten area, certain populations are still declining, while others are stable or increasing.
For the Barents Sea–Lofoten area as a whole, the sectors that are most important in driving environmental change have been identified as the fisheries, transport, and oil and gas, but aquaculture, coastal infrastructure, wastewater treatment and agriculture also play a role. They are responsible for a range of pressures, some of the most important being selective species extraction, abrasion, pollution, underwater noise and smothering.
3.1.1 Ecosystem condition in the Barents Sea–Lofoten management plan area
Ecosystem condition has been assessed separately for the Arctic (northerly) and subarctic (southerly) parts of the Barents Sea, limited to Norwegian waters. Based on long time series starting around 1970, the scientific panel for the Barents Sea has concluded that anthropogenic drivers have had substantial impacts on the climate and the physical environment in these ecosystems, particularly through rising temperatures and declining sea ice cover. In contrast, the biological components of the ecosystem have largely been assessed on the basis of data for the period 2004–2020. As a result, the impacts of warming up to 2004 are uncertain. Nevertheless, there is evidence of limited human impact on the Arctic ecosystem as a whole. For the Arctic part of the Barents Sea, the observed changes include a weak tendency for primary production to increase and algal blooms to take place earlier in spring, and a tendency for the food web to change, with a decline for both top predators and seabirds. Nevertheless, important ecosystem functions appear to have been maintained. A decline in Arctic fish species was observed up to about 2015 but has been reversed to some extent in recent years, when temperatures have been lower.
For the subarctic part of the Barents Sea, changes in the physical environment have been observed as a result of climate change and fisheries pressure. Apart from this, there is not currently any evidence of impacts on the ecosystem as a whole, but climate change is expected to have impacts on the biological ecosystem components as well in the years ahead. However, there is considerable uncertainty associated with these conclusions because the time series for the biological components are so short. It is therefore also very uncertain whether the impacts really are limited or whether more extensive impacts have not been demonstrated because there are important indicators that were not included in the assessment and many of the time series are too short.
Fisheries pressure appears to have diminished in recent years. The assessment of ecosystem condition includes three indicators for benthic fauna that may be affected by bottom trawling. For the period 2004–2020, there is no sign of major changes in these indicators that can be linked to bottom trawling, but there is uncertainty associated with the assessment.
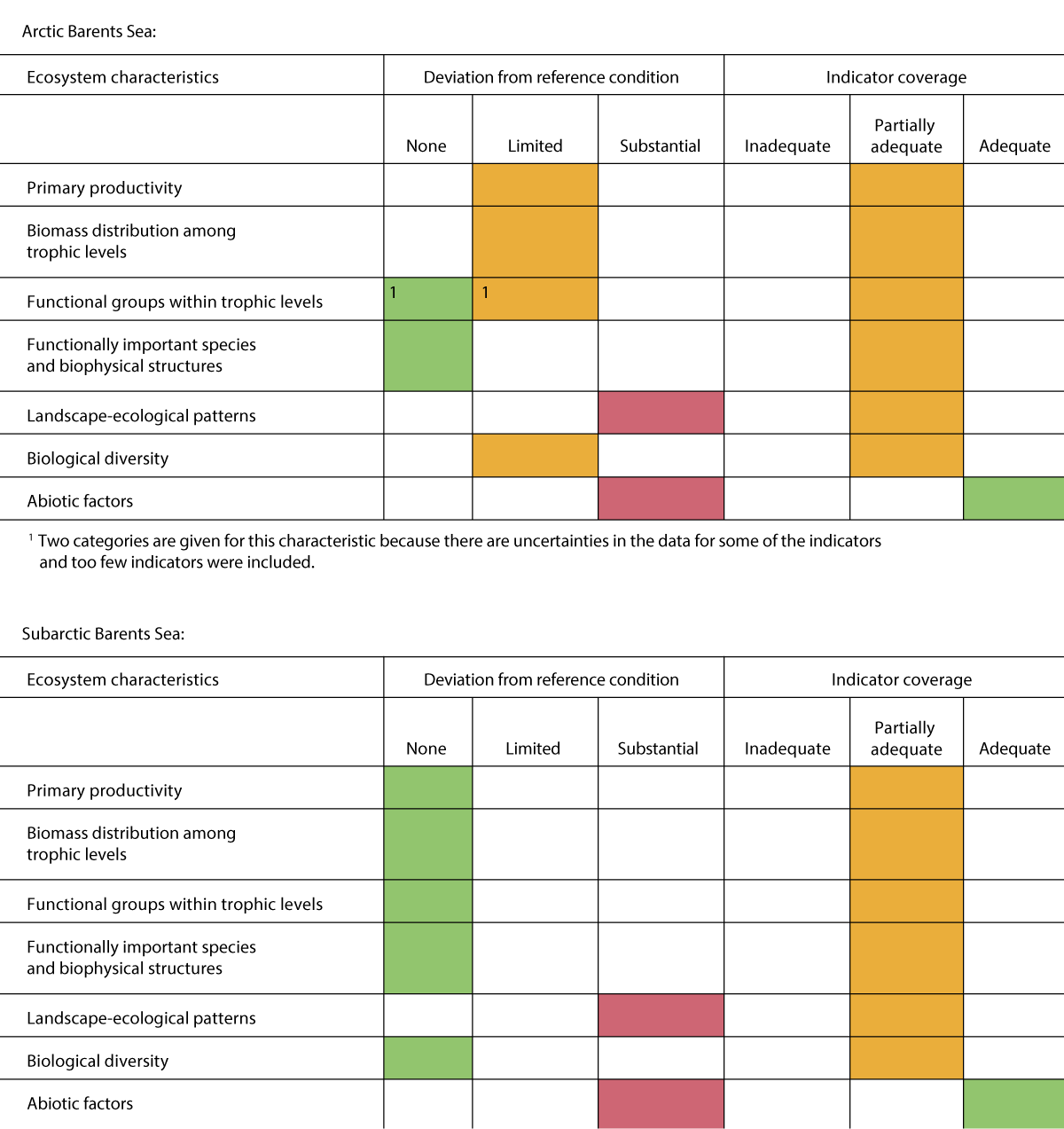
Figure 3.2 Summary of the assessment of ecosystem condition for each of the ecosystem characteristics. Upper panel: Arctic part of the Barents Sea. Lower panel: subarctic part of the Barents Sea.
Source: Panel-based Assessment of Ecosystem Condition of Norwegian Barents Sea Shelf Ecosystems. Rapport fra havforskningen 2023-14.
The ecological status of most of the coastal water bodies in the Barents Sea is classified as good according to the system used in the Water Management Regulations. There are only a few areas of fjords that are classed as of moderate status, and one is classed as poor. The biological quality elements used to classify the ecological status of coastal water bodies are soft-bottom benthic invertebrates, eelgrass, macroalgae, and phytoplankton, and in addition there are hydromorphological and physico-chemical quality elements.
3.1.2 Oceanic climate change: Barents Sea–Lofoten management plan area
The ocean climate in the Barents Sea–Lofoten area is to a large extent determined by the temperature and volume of Atlantic water that flows into the area from the Norwegian Sea. Ocean climate variables are water temperature, salinity, ocean currents, ocean acidification and sea level.
In the Barents Sea, there has been an average rise in sea temperature of about 1.5 °C over the past 50 years. Recently, a certain decline in sea temperature has been observed, coinciding with a reduction in the inflow of Atlantic water from the Norwegian Sea (Figure 3.3), but the temperature now appears to be rising again.
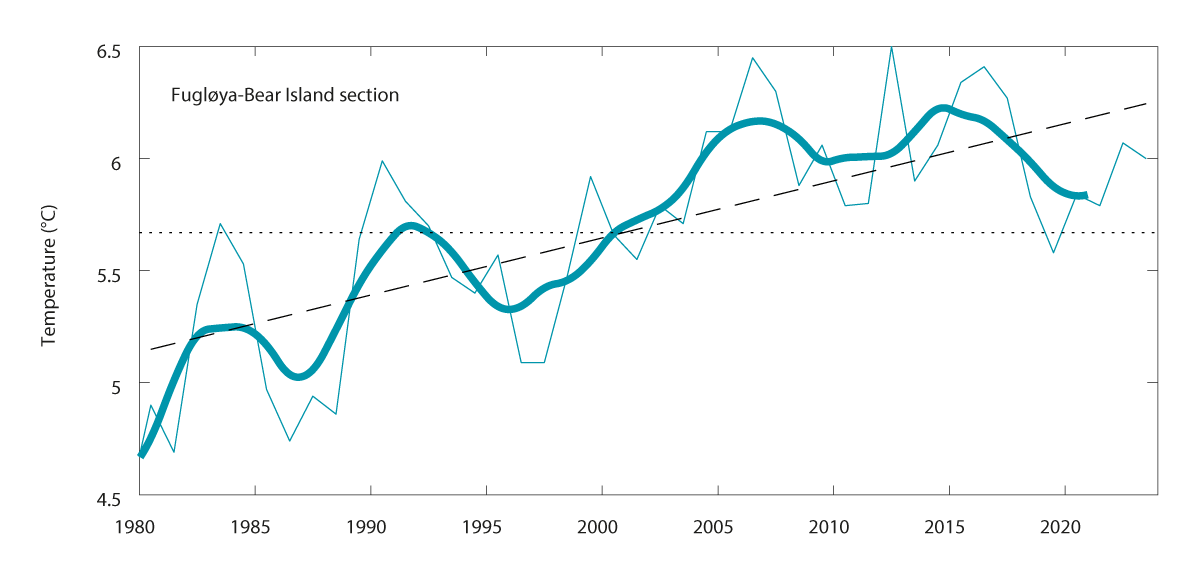
Figure 3.3 Temperature averaged within the depth interval 50-200 m, in the core of Atlantic water for the Fugløya-Bear Island section. The narrow line shows annual means, the bold line shows a smoothed curve using five-year means, and the dashed line shows the linear trend.
Source: Institute of Marine Research
In addition, the climate in the northern Barents Sea is strongly influenced by the extent of the sea ice. Climate change has resulted in a decline in sea ice in the Barents Sea over the past 40 years (Figure 3.4). Snow-covered sea ice can reflect up to 80 % of incoming solar energy, whereas open sea absorbs 90 % of the energy. Warming in the Arctic that causes sea ice to melt is amplified because this results in the absorption of more energy and further Arctic warming.
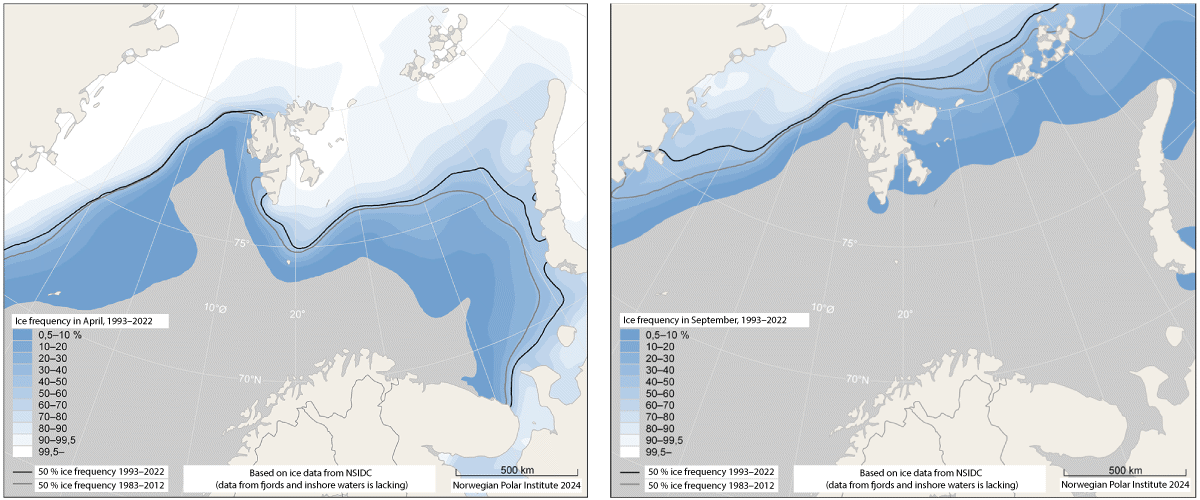
Figure 3.4 Sea ice extent in the Barents Sea and Fram Strait. The maps show the situation in April, when sea ice extent normally reaches its annual maximum, and September, when it is at a minimum. Sea ice extent is shown as ice persistence, based on a time series of satellite observations for the 30-year period 1992-2022. Ice persistence is a measure of how often at least 15 % of the water surface is covered by ice in a specific period within a specific area. The grey and black lines show the change in sea ice extent in April and September from the period 1983-2012 (grey line) to the period 1993-2022 (black line). The lines show where there was ice for half of each of the two months in the relevant 30-year period.
Source: Norwegian Polar Institute
The degree of ocean acidification (Box 3.2) varies widely across the Barents Sea, largely as a result of variations in water masses, biological production and ice formation. In the past 20 years, CO2 levels have risen rapidly, particularly in parts of the Barents Sea where there is seasonal ice cover, where the rise is twice as large as the global trend. This has been linked to shrinking ice cover. In the southern Barents Sea, which is most strongly influenced by southerly water masses (Atlantic water), the rate of ocean acidification appears to be following the global trend.
Textbox 3.2 Ocean acidification
The oceans absorb about 30 % of anthropogenic CO2 emissions to the atmosphere. As a result, the CO2 concentration in the oceans is rising. When CO2 reacts with water, it forms carbonic acid, which releases hydrogen ions. This results in lower pH and ocean acidification. Some of the hydrogen ions react with carbonate ions in the seawater to form bicarbonate, which makes carbonate less accessible to living marine organisms. Carbonate is essential for organisms that build calcareous shells and skeletons consisting of calcium carbonate. These include the sea snail Limacina helicina, bivalves and cold-water corals. Such calcifying organisms may meet serious problems as carbonate levels drop. Their shells or skeletons may begin to dissolve, or they may need more energy to build the shell or skeleton. Ocean acidification has been monitored in Norwegian waters since 2010. Arctic seas are particularly vulnerable to ocean acidification, because cold water can absorb more CO2 than warmer water. The impacts of ocean acidification on ecosystems are very uncertain, but are potentially serious.

Figure 3.5 Sea snail (or 'sea butterfly') Limacina helicina
Photo: Erling Svensen
Climate change has resulted in rising temperatures, ocean acidification and melting of the sea ice in the Barents Sea–Lofoten area. Because the time series are relatively short, the impacts on the ecosystems are uncertain. However, further warming of the climate is expected in the future, and is likely to result in large-scale changes to the ecosystems in the area.
Observed effects of changes in ocean climate
Seawater temperatures influence primary production in the oceans and the distribution of many species, and thus species composition in particular areas. In the Barents Sea, there is a weak tendency both for primary production to increase and for algal blooms to occur earlier in spring. A considerable increase in quantities of krill (which belong to the zooplankton) has been observed in parallel with the warming of the Barents Sea. The previous white paper on the management plans described how Atlantic and more southerly fish species are shifting their distribution northwards and eastwards from the southwestern part of the Barents Sea, and displacing Arctic species such as polar cod (Boreogadus saida). This trend has been reversed as a result of the recent decline in sea temperature.
Most seabird species are showing a negative population trend, which in many cases can be explained by food shortages combined with the impacts of climate change. Changes in food supplies may be the result of a combination of lower production of prey species, natural competition for food, fisheries, and secondary effects of climate change. Warming seas influence zooplankton species composition, for example different Calanus species in the North Sea (see Chapter 3.3.2). In recent years, mackerel have been observed much further north in the Barents Sea. Their diet includes large quantities of zooplankton and small fish, and mackerel may therefore be important as competitors for food with seabirds. Climate change may also have direct impacts on seabird populations, since more frequent extreme weather events can affect both survival and breeding success. Changes in climatic conditions in turn influence oceanographic conditions, which determine the availability of food such as fish larvae for breeding seabirds. As a result, the timing of maximum food availability may shift, leading to a mismatch between the peak prey availability and the seabird breeding season, which often does not shift correspondingly.
Climate change is now generally the most important driver of change for Arctic marine mammals. Blue whales and fin whales, which are subarctic species, are shifting their distribution northwards as the sea ice retreats.
3.1.3 Condition and trends for various ecosystem components in the Barents Sea–Lofoten area
The condition and main trends for various ecosystem components in the Barents Sea–Lofoten area are described below using the results of the assessment of ecosystem condition for the management plan area.
Plankton
In the Arctic part of the Barents Sea, there is some evidence that annual primary production is rising and that the spring algal bloom is starting earlier in the year.
The benthic fauna and benthic communities
New benthic species (crustaceans, sponges, seapens, corals, etc) are constantly being identified during the annual Norwegian-Russian ecosystem surveys in the Barents Sea. Over time, the largest biomass quantities have been observed in the northeastern part of the Barents Sea, followed by the southwestern, northwestern and southeastern parts. Results for the period 2006–2021 show a moderate positive trend in the biomass of megabenthic species. Several types of benthic communities (for example soft-bottom sponge aggregations, coral gardens and sublittoral seapen communities) are vulnerable to human pressures such as bottom trawling and other activities that involve contact with the seabed.
The snow crab (Chionoecetes opilio) is widely distributed in open sea areas of the Barents Sea. The distribution of the stock on the Norwegian continental shelf has not changed significantly in recent years. New genetic analyses indicate that snow crabs spread naturally to the Barents Sea, and it has therefore been removed from the list of alien species maintained by the Norwegian Biodiversity Information Centre. At present, the snow crab does not appear to have the same negative impacts on benthic communities in the Barents Sea as the red king crab (Paralithodes camtschaticus). The westward spread of snow crabs in the Barents Sea is expected to continue. Bottom trawling has major impacts on the seabed, especially on habitats for organisms such as corals, sponges and seapens. The extent to which bottom trawling has resulted in changes to the ecosystem is uncertain. The most intensive bottom trawl fisheries in the Barents Sea are along the coast of Finnmark, in the southeastern part of the Norwegian sector, around Bjørnøya (especially south of the island), and south of Spitsbergen. There may be interest in bottom trawling in areas that become accessible as the ice retreats, but strict management rules have been established to limit the expansion of the fisheries to previously ice-covered areas.
Fish stocks
The major fish stocks play a key role in ecosystem dynamics and are important in economic terms. Following the introduction of more sustainable fishing practices, there was a period when the major commercial fish stocks (cod, haddock, capelin, beaked redfish and saithe) showed a positive development trend. More recently, the cod and haddock stocks have declined, but their spawning stocks are still above the precautionary level. The Greenland halibut stock is just below the precautionary level, while the golden redfish is endangered, and the stock is declining and is at a critically low level. Fishing mortality (the harvest) is too high for cod, Greenland halibut and golden redfish. The shrimp stock is healthy.
Capelin and polar cod are key species in the Barents Sea ecosystem throughout the year. The capelin stock is showing a positive trend. The polar cod stock is endangered, and has been declining since 2000, but showed an upturn in 2020 and 2021. The quantity of young herring in the Barents Sea is currently at a low level.
Apart from climate change, the fisheries are the most important driver in the Barents Sea–Lofoten area. Pressure from the fisheries has generally been reduced since 2000 through the introduction of management measures and developments in fishing gear.
Seabirds
Populations of most seabird species in the Barents Sea are still declining, but there are positive indications for some populations and breeding colonies. Certain gannet, fulmar, great skua and common guillemot colonies are growing. The outbreak of avian flu early in the 2022 breeding season appears to have hit the gannet and great skua populations hardest, and this is expected to slow or reverse the positive trend for these two populations. The kittiwake population suffered badly from the avian flu outbreak in 2023. The populations of Brünnich’s guillemot, kittiwake, razorbill and shag are still declining (Figure 3.6). Many of the seabird species in the Barents Sea–Lofoten area are on Norway’s red list. Regional estimates of seabird populations will be updated in 2025.
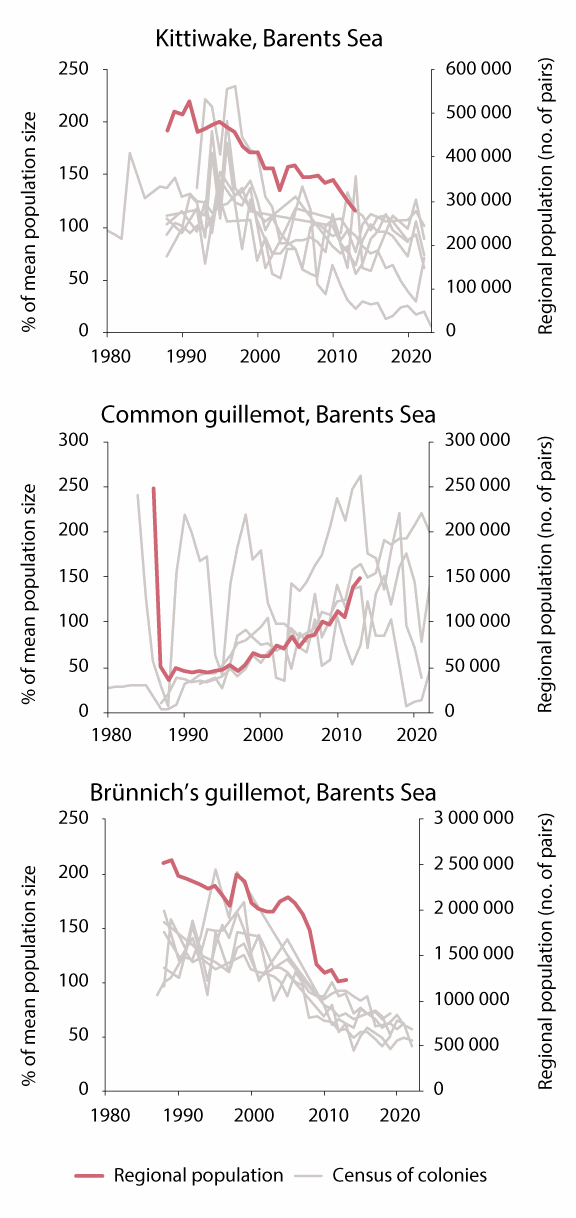
Figure 3.6 Seabird populations in the Barents Sea. Population trajectories for kittiwake, common guillemot and Brünnich's guillemot. Bold red lines show the estimated population size, thin grey lines show figures from monitoring at specific colonies.
Sources: Regional population figures from NINA Report 1151 (2015), monitoring data from seapop.no
In addition to climate change and food shortages, drivers for seabird populations may include hazardous pollutants and plastic pollution. High levels of persistent, bioaccumulative and toxic substances have been measured in seabird eggs, including two Arctic species, glaucous gull and ivory gull. Such pollutants may have various impacts on seabirds, for example impaired reproduction, skewed sex distribution in offspring and higher mortality. The growing white-tailed eagle population is having a negative impact on cliff-nesting birds such as common guillemot and kittiwake.
Marine mammals
Populations of some marine mammals in the Barents Sea are at low levels as a result of historical whaling operations, but are now growing after being protected (for example walrus, hooded seal, polar bear and blue whale). Numbers of several whale species have increased along the coast of Svalbard. Harp seals and minke whales are currently harvested in the Barents Sea, and their populations are in good condition. Sealing operations are also carried out in Svalbard. Marine mammals are top predators, and accumulate hazardous substances in their tissues. There is concern, for example, about high levels of persistent, bioaccumulative and toxic substances in polar bears and orcas. A number of whale species communicate over long distances by means of sound, and noise from shipping, seismic activities and sonar may have negative impacts on these species.
The Arctic marine mammals in the Barents Sea are expected to be adversely affected by climate change. The impacts may be direct, through the loss of sea ice for species that are dependent on the ice as a habitat (ringed seal, bearded seal, harp seal, hooded seal, bowhead whale, narwhal, beluga, polar bear), or indirect, as a result of changes in food supplies. Climate change is expected to have a positive impact on the subarctic species blue whale and fin whale through higher pelagic biological production, which is predicted to be a result of shrinking sea ice cover. The increase in krill biomass that has been observed in parallel with the warming of the Barents Sea may be particularly important. Humpback and minke whales are expected to be able to adapt to changes in the food web. Rising temperatures and the loss of sea ice are expected to result in a northward shift in the distribution of various prey species, and both humpback and minke whales are therefore expected to expand their distribution northwards. Continued warming is expected to result in an increase in orca numbers, and if important prey species such as mackerel and herring spread northwards, orcas are expected to follow suit.
Alien species
Species that are introduced outside their natural range through human agency are considered to be alien species. Alien species can alter the natural species composition in an area and thus the local ecosystem. With only a few exceptions, alien species in Norwegian coastal and marine waters are not systematically monitored.
There are few alien species in the Barents Sea. The most important are the red king crab and pink salmon (Oncorhynchus gorbuscha), and the distribution of both species is being monitored. The king crab is found mainly in the large fjords in eastern Finnmark, and is not as numerous in open waters along the coast. There has been little change in the population size and distribution of red king crab in recent years. Studies show that in areas where king crabs have been present in large numbers for a long time, the species has substantial effects on benthic ecosystems. At the same time, the species is a valuable resource for the fisheries industry. In coastal waters and fjords east of North Cape, harvesting of king crabs is regulated by quotas. In coastal waters west of North Cape, harvesting is unrestricted to attempt to limit the westward spread of the species. Individual crabs are regularly observed in several areas in western Finnmark and in Troms, and especially around Tromsø, where they are frequently observed and caught (Figure 3.7).
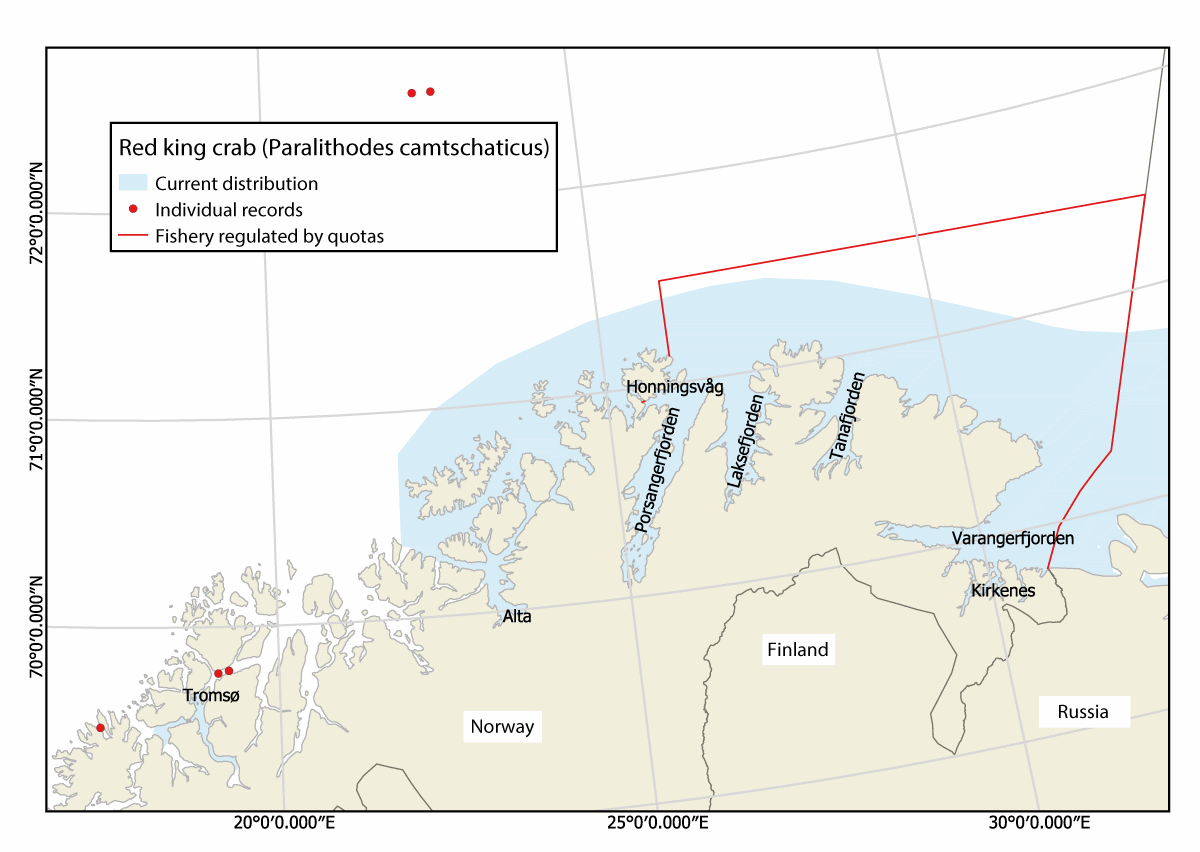
Figure 3.7 Current distribution of red king crab in the Barents Sea (both the Norwegian and the Russian sectors of the continental shelf), and individual records.
Source: Institute of Marine Research
Pink salmon first appeared in rivers along the Norwegian coast in 1960. The species is originally from the Pacific Ocean, but was introduced in rivers on the Atlantic side of Russia, and has since spread to Norwegian rivers. Pink salmon numbers in the North Atlantic remained very low for many years. The species has a two-year life cycle, and the population that spawns in odd-numbered years has exploded from 2017 onwards. In these years, pink salmon is now the most numerous salmonid species in rivers in Troms and Finnmark. The rise in sea temperatures in northern waters has probably played an important role in the increase in pink salmon numbers. Pink salmon poses a serious risk for wild Atlantic salmon and biodiversity in general. Since all pink salmon die in the rivers after spawning, there is also a risk to water quality. In both 2021 and 2023, considerable efforts were made to remove pink salmon from rivers in Troms and Finnmark. In 2023, action was taken in more than 90 rivers, and more than 99 000 pink salmon were caught in the sea and 243 000 in the rivers.
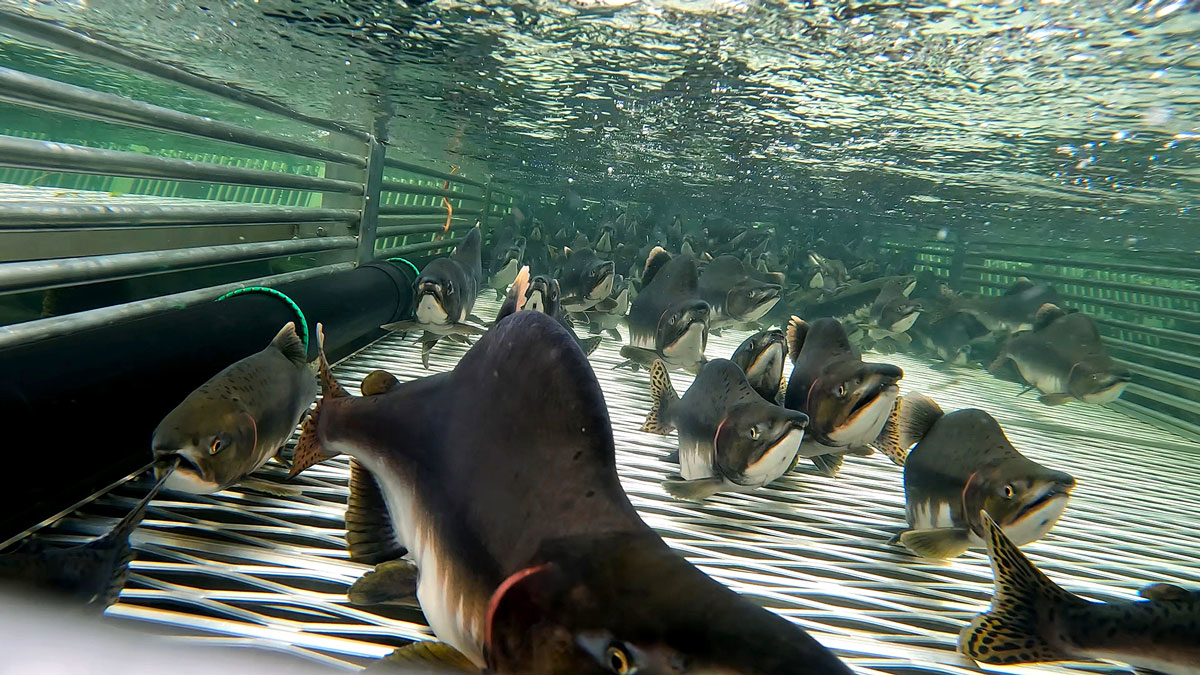
Figure 3.8 Pink salmon (Oncorhynchus gorbuscha) caught in a trap.
Photo: Malin Solheim Høstmark, Office of the County Governor of Troms og Finnmark
Threatened species and habitat types
The Norwegian red lists of habitat types and species were updated in 2018 and 2021 respectively. There has been a clear deterioration in the situation for threatened species and habitat types in Norway’s marine and coastal waters. In all, 45 species in the Barents Sea–Lofoten area, including Svalbard, are on the 2021 Norwegian red list, and 39 of these are listed as threatened (in one of the categories critically endangered, endangered or vulnerable). Conservation status is poorer for 18 species and better for six species than in the 2015 red list. Species that are now considered to be critically endangered or endangered include mammals (bowhead whale, beluga and hooded seal), nine bird species (including black-headed gull, puffin, Brünnich’s guillemot and common guillemot) and seven fish species (including basking shark, the skate Dipturus intermedius, golden redfish and polar cod). Overall, changes in conservation status are assessed as most negative for seabirds.
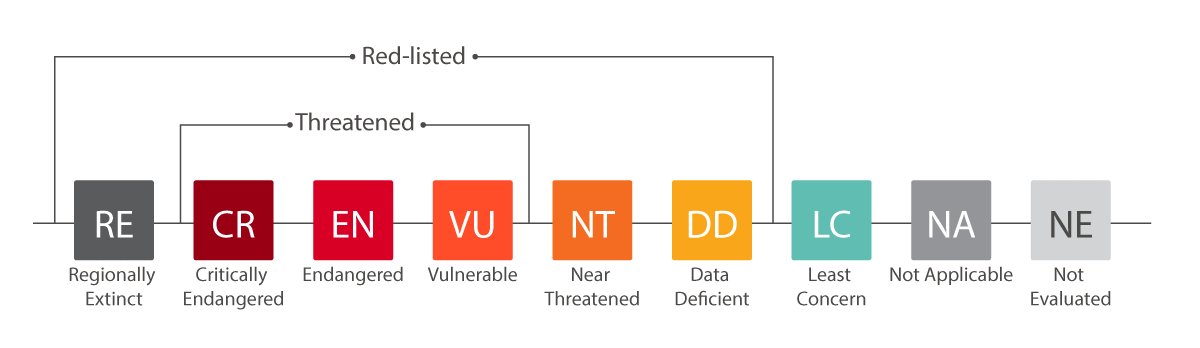
Figure 3.9 Red list categories for species.
Source: Norwegian Biodiversity Information Centre
Only two habitat types in the Barents Sea were assessed as threatened in the 2011 red list, but eight more were added in the 2018 red list. The conservation status of Radicipes coral gardens deteriorated from vulnerable to endangered, while coral reefs showed an improvement, from vulnerable to near-threatened. Arctic sea ice is classified as critically endangered, and northerly sugar kelp forests as endangered.
3.1.4 Pollution
Pollution levels are generally lower in the Barents Sea–Lofoten area than in the other two management plan areas. Long-range transport of hazardous substances with ocean currents and in the atmosphere is the main source of pollution in the Barents Sea, while inputs from rivers are also an important source of mercury. Otherwise, there are few local sources of pollution in the management plan area. Discharges of produced water (oily water from the oil reservoirs) and of chemicals from oil and gas activities in the area are small. Aquaculture facilities along the coast are another source of pollution.
For many years, the quantities of various persistent, bioaccumulative and toxic substances that are transported to the Barents Sea–Lofoten area with ocean currents and in the atmosphere have been declining, but this reduction has stagnated in recent years. Levels of certain substances, including chlorinated pesticides such as hexachlorobenzene (HCB), increase from south to north in biological samples, and the highest levels are therefore found in the Barents Sea.
Persistent, bioaccumulative and toxic substances, particularly those that are fat-soluble, accumulate along food chains and have particularly serious impacts on top predators. Research indicates that these chemicals are having impacts on seabirds and marine mammals in the Barents Sea–Lofoten area. Starving polar bears may be particularly vulnerable to the effects of these pollutants. However, seafood from Norwegian waters is safe to eat, since concentrations of contaminants are generally below the maximum permitted levels for food safety in edible parts of most species.
Levels of man-made radioactive substances in the Barents Sea are low. Levels of caesium-137 (originating from the Chernobyl accident), which are measured in sea water, seaweed and several fish species, are low and sinking. Concentrations of man-made radioactive substances are low, and below the maximum levels for human consumption.
There has been a steep rise in inputs of phosphorus, nitrogen and copper to the coastline bordering the Barents Sea–Lofoten area since 1990 (Figure 3.10). There are inputs from various sources, but fish farming is responsible for most of the large increase in inputs. The use of copper for impregnation in the aquaculture industry has been reduced in recent years. Little is known about how nutrients are transported from coastal waters to the open sea. It is therefore unclear what proportion of nutrient inputs is transported from coastal waters into the management plan area.
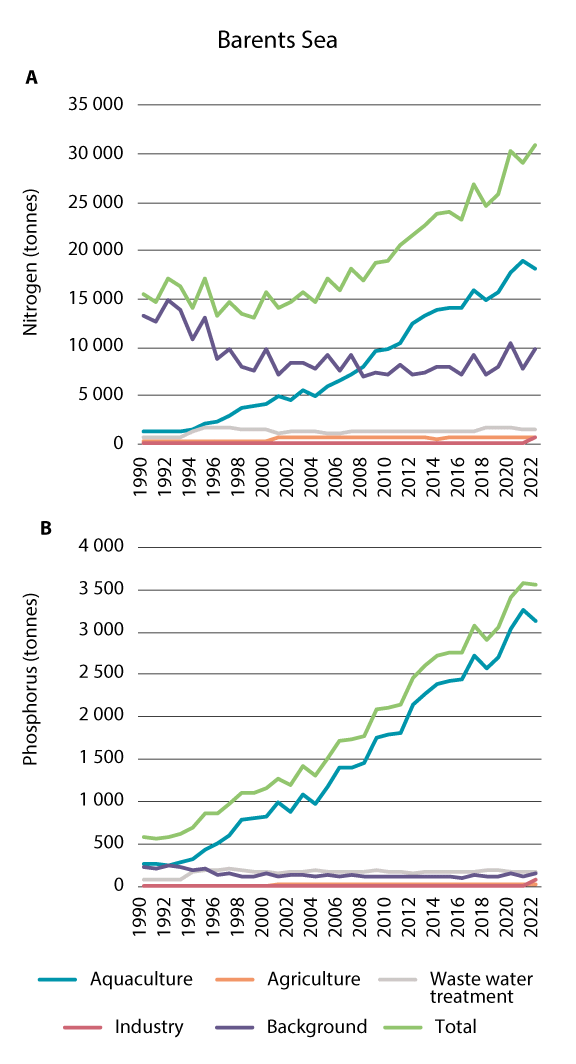
Figure 3.10 Inputs of nitrogen (a) and phosphorus (b) to the coastline bordering the Barents Sea–Lofoten area in the period 1990-2022.
Source: NIVA report 7963-2024
Fisheries-related waste, including lost fishing gear, is a major source of marine litter and plastic pollution in the Barents Sea–Lofoten area. Most floating waste is of relatively local origin, but waste can also be transported from distant waters. Little is known about trends for marine litter and plastic pollution in this management plan area. Marine litter has been monitored on four beaches along the border of the management plan area, two in Svalbard, one in Tromsø and one in Finnmark. Monitoring shows continued inputs of marine litter, but the data are not sufficient to draw conclusions about trends over time. Knowledge will be built up through national monitoring programmes for microplastics and macroplastics and more intensive monitoring of beach litter under OSPAR.
3.2 Ecosystem condition, drivers of change and impacts in the Norwegian Sea management plan area
As in the Barents Sea–Lofoten area, the impacts of climate change are becoming apparent in the Norwegian Sea management plan area, in the form of rising temperatures, shrinking sea ice cover and signs of ocean acidification. The fisheries are an important driver in the Norwegian Sea as well. The stocks of mackerel and Norwegian spring-spawning herring are declining, while the stocks of Northeast Arctic saithe and beaked redfish are at high levels. The golden redfish stock is declining and has fallen below the critical level. Seabird populations in the Norwegian Sea are still declining steeply, but improvements have been observed at certain colonies.
There are signs that southerly zooplankton species that prefer higher water temperatures are shifting their distribution northwards into the Norwegian Sea.
Inputs of hazardous substances with air and ocean currents are generally declining. Levels of contaminants in seafood are generally low and below the maximum permitted levels for food safety. However, there are some exceptions, and the authorities have either taken steps to prevent the relevant species from reaching the market or issued advisories against consumption. Inputs of phosphorus, nitrogen and copper to coastal waters have risen a great deal in recent years. It is unclear what proportion of discharges to coastal waters is transported into the management plan area.
3.2.1 Ecosystem condition in the Norwegian Sea management plan area
In the Norwegian Sea, ecosystem condition has only been assessed for one of the eleven ecosystems identified in the area: the pelagic ecosystem south of the Arctic front. This includes the upper 800 m of the water column in the deeper parts of the Norwegian Sea. For most of the other ecosystem types in the Norwegian Sea, little or no monitoring data is available.
There is evidence that human activities are having limited impacts on the pelagic ecosystem in the Norwegian Sea (see Figures 3.1 and 3.11). The clearest climate-related change is the temperature rise that has been observed over the past 70 years. There are also signs of increasing ocean acidification, but the biological consequences of this are very uncertain. In addition, stocks of mackerel and Norwegian spring-spawning herring are declining. This is because catches have been far higher than the recommended TACs (total allowable catches) after the international system for sharing the TACs collapsed in 2013. The herring stock has declined so much that advice has been issued for 2024 that fishing pressure must be reduced to avoid a decline to below the critical level in the long term.
The substantial decline that has been observed in seabird populations has been linked to rising sea temperatures in the Norwegian Sea and to changes in food supplies. For other ecosystem components than seabirds, no observed changes in the indicators used for this assessment can reasonably certainly be attributed to climate change. It is very uncertain whether the impacts really are limited or whether there are more serious impacts that have not been demonstrated because important indicators were not included in the assessment and many of the time series are too short.
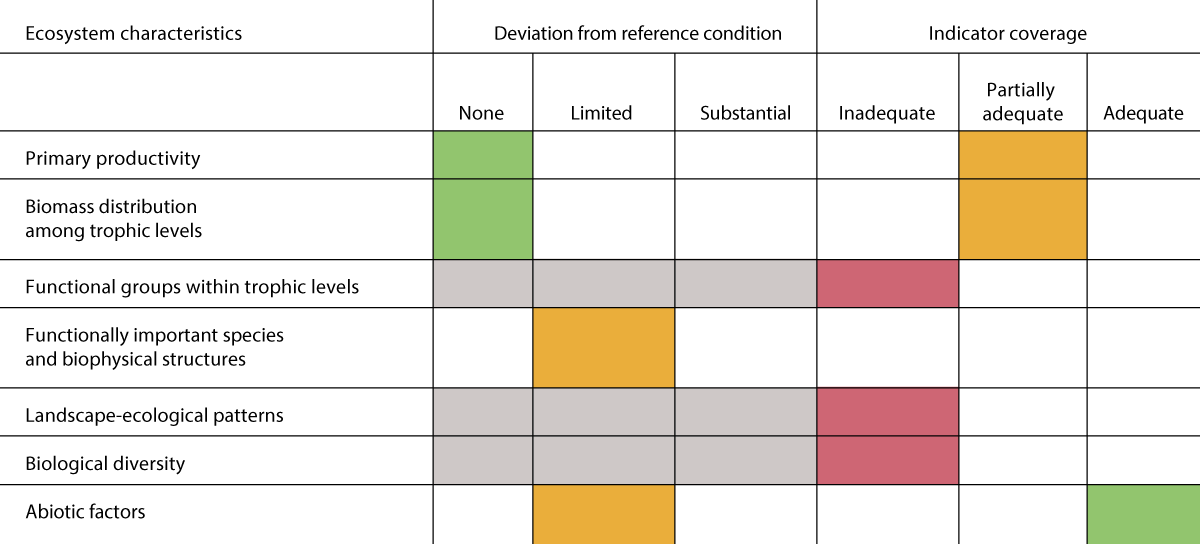
Figure 3.11 Summary of the assessment of ecosystem condition for each of the ecosystem characteristics for the Norwegian Sea pelagic ecosystem. Grey cells indicate that no information is available.
Source: Panel-based Assessment of Ecosystem Condition of the Norwegian Sea Pelagic Ecosystem. Rapport fra havforskningen 2023-16.
The ecological status of about 80 % of the coastal water bodies in the Norwegian Sea is classified as good, and in some cases high, according to the system used in the Water Management Regulations. About 17 % are classed as of moderate status, and only a few water bodies are classified as bad. The biological quality elements used to classify the ecological status of coastal water bodies are soft-bottom benthic invertebrates, eelgrass, macroalgae, and phytoplankton, and in addition there are hydromorphological and physico-chemical quality elements.
3.2.2 Oceanic climate change: Norwegian Sea management plan area
The ocean climate in the Norwegian Sea is closely linked to the characteristics of the Atlantic water that flows into the area from the south with the Norwegian Atlantic current. Long-term variations in the Norwegian Sea will therefore follow general climate trends in the North Atlantic, but with a few years’ delay. In addition, interannual variations in the Norwegian Sea are strongly influenced by variability in heat loss from the sea to the atmosphere and fluctuations in the inflow of relatively cold, fresh Arctic water from the Iceland and Greenland Seas. Because the time series are relatively short, the impacts of climate change on the ecosystem are uncertain.
The Norwegian Sea has become generally warmer in the last 40 years (Figure 3.12), and this can be clearly linked to anthropogenic climate change. The temperature rose by 1 °C from 1980 to about 2005. Since then, the Norwegian Sea has been relatively warm despite an increase in the inflow of relatively cold Arctic water from the west.
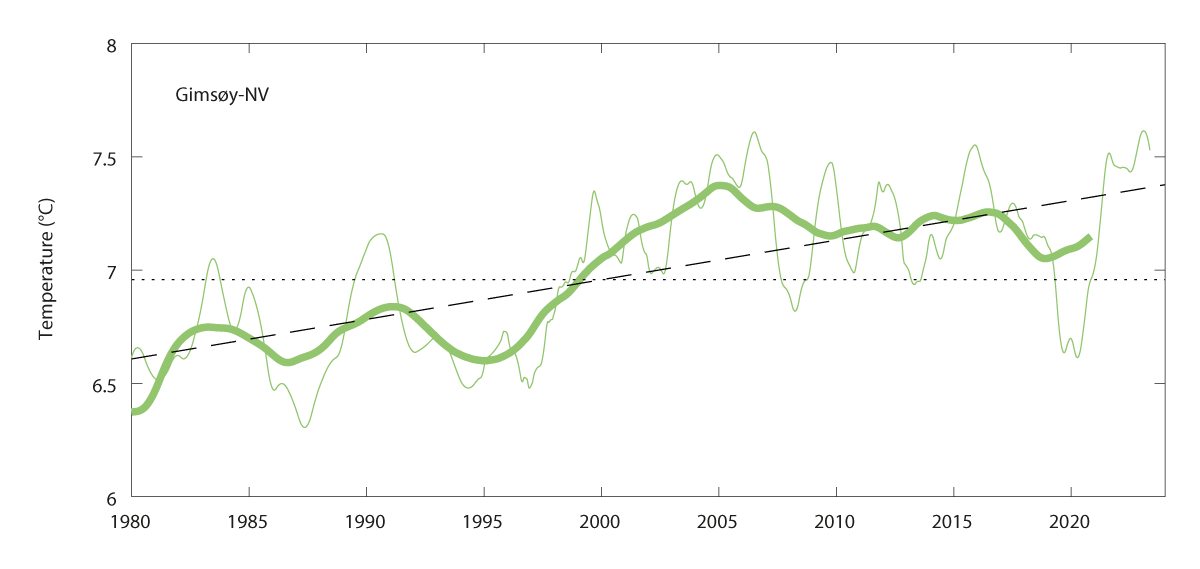
Figure 3.12 Temperature for the period 1980-2020 averaged within the depth interval 50-200 m, in the core of Atlantic water for the Gimsøy section (northern part of the Norwegian Sea, west of the Lofoten Islands). The narrow line shows annual means, the bold line shows a smoothed curve using five-year means, and the dashed line shows the linear trend.
Source: Institute of Marine Research
There is sea ice in the Fram Strait in the northern part of the Norwegian Sea. Higher sea and air temperatures are reducing sea ice extent. There are large interannual variations, but also a clear negative trend for sea ice extent in the Fram Strait.
There are signs of increasing ocean acidification in the Norwegian Sea. In the Norwegian Basin, pH in the surface waters has fallen by 0.12 units over the past 40 years. It appears that pH is decreasing more rapidly in parts of the Norwegian Sea than at global level. Changes in aragonite saturation are also significant because this carbonate mineral is very important for species with calcium-based shells or skeletons (see Box 3.2). The observed changes in pH and aragonite saturation level in the Norwegian Sea are largely explained by the higher CO2 content of the water as a result of CO2 emissions to air from human activity. Changes in the water masses or inputs of freshwater also add to ocean acidification.
Observed effects of changes in ocean climate
Several copepod species and Cymbulia peronii, a pelagic sea snail, are examples of southerly warm-water zooplankton species that have been observed in the Norwegian Sea. A sharp increase in southerly species was observed in the area from 2006 onwards. Since 2011, there has been a general decline, but with wide interannual variations. Changes in the occurrence of southerly species may be linked to higher sea temperatures. These are partly explained by an increase in water transport from the North Atlantic, which is one factor behind the northward shift in the distribution of more southerly species.
The severe decline in seabird populations has been linked to factors including rising water temperatures in the Norwegian Sea. Climate change also influences where and when fish spawn and the survival of young fish. This can cause a mismatch between the availability of fish larvae and the breeding cycle of seabirds, disrupting seabird reproduction. Thus, rapid climate change is having negative impacts on the productivity of pelagic seabirds that breed in colonies around the Norwegian Sea management plan area, and this mechanism is partly responsible for the current decline in seabird populations.
3.2.3 Condition and trends for various ecosystem components in the Norwegian Sea
The condition and main trends for various ecosystem components in the Norwegian Sea pelagic ecosystem are described below using the results of the assessment of ecosystem condition.
Plankton
No changes have been observed in primary production (phytoplankton) in the Norwegian Sea in the past 20 years. The time series for zooplankton are too short to assess whether warming has resulted in important changes for this group, as has been observed in other areas where longer time series are available.
Benthic communities
The MAREANO programme for mapping of the seabed has been collecting data on the benthic fauna in the Norwegian Sea since 2011 (Figure 3.13). Benthic animals may have a scattered distribution or be found in dense stands. A number of benthic communities, such as hard- and soft-bottom coral gardens, coral reefs, hard-bottom sponge aggregations and seapen communities, are vulnerable for example to abrasion. Biodiversity is highest in areas of the continental shelf with a sloping seabed and in the upper parts of the continental slope, for example in coral reef complexes such as Røstbanken, the Storegga area and the Møre banks.
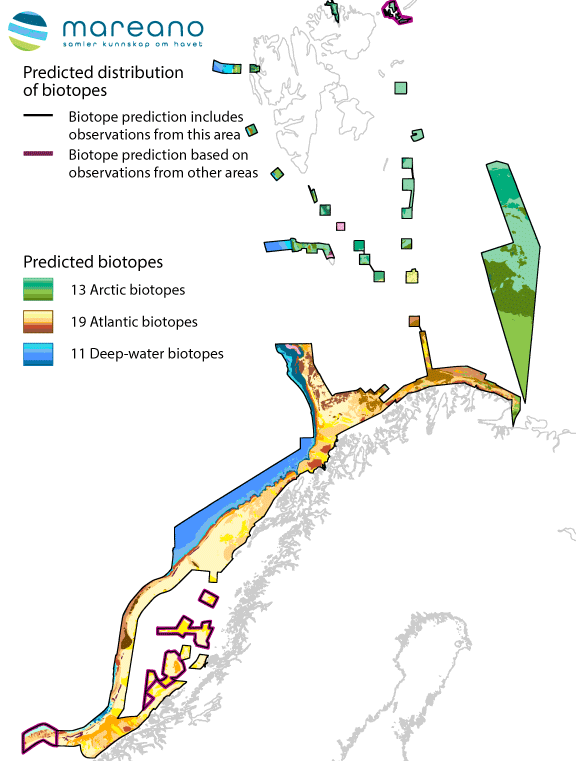
Figure 3.13 General biotope map showing the predicted distribution of benthic biotopes in the Norwegian Sea and Barents Sea.
Source: MAREANO programme
Kelp forests are considered to be particularly productive ecosystems with a high level of species diversity. They function as important nursery areas and shelter for fish larvae, and also play a role in carbon storage. Northerly sugar kelp forests along the coast of the Norwegian Sea are endangered by intense grazing pressure from sea urchins, probably because species that are sea urchin predators (wolf-fish, haddock and cod) were overfished up to the 1970s. However, crabs are expanding northwards with rising temperatures, and this appears to be having a positive impact on kelp forests, since crabs feed on sea urchins and reduce their numbers.
The largest kelp forests are formed by Laminaria hyperborea, and there are particularly extensive stands along the coast of Møre og Romsdal and Trøndelag counties. Given its temperature preference, the distribution of L. hyperborea is expected to remain stable in southerly coastal waters and expand somewhat further north in the coming decades. Kelp forests are a renewable resource that can be harvested sustainably along the coast. Kelp trawling can have major impacts on the flora and fauna in and around kelp forests. After harvesting, the physical structure and age distribution of kelp forests change and become more uniform. Harvesting is permitted every five years, by which time the biomass of kelp has recovered. However, it may take longer than this before a re-established kelp forest is ‘ecologically mature’ and all ecosystem functions have recovered.
Fish stocks
Fishing pressure on mackerel, herring and blue whiting has been higher than recommended in recent years. This is largely because no binding international agreement has been reached on sharing these fisheries in the Norwegian Sea since 2013. The mackerel stock is nevertheless in relatively good condition, and recruitment is strong. Since 2008, the spring-spawning herring stock has been declining, largely because of weak recruitment. The most recent strong year class was observed in 2016. Harvesting above recommended TACs weakens the stock, which in 2024 is expected to fall below the level at which further management measures are needed to prevent it from reaching a critically low level. Over the long term, the blue whiting stock is becoming stronger. Even though catches are higher than the recommended TAC, strong recruitment has ensured that the stock is resilient in recent years. The stock of Northeast Arctic saithe was close to a historically high level in 2022.
The other commercial fish stocks are healthy – these are beaked redfish, Greenland halibut, tusk, ling, spiny dogfish and greater argentine. Stocks of golden redfish and blue ling are low, and both species are endangered. Catches of these species and of three elasmobranches, the skate Dipturus intermedius, basking shark and porbeagle, are prohibited. The stocks of coastal cod are in poor condition.
It is possible that there will be interest in expanding Norwegian Sea fisheries for species at lower trophic levels, such as the copepod Calanus finmarchicus. This would be one way of providing feed for the growing aquaculture industry. Potential problems such as overharvesting, bycatches of other species and impacts on the structure of ecosystems will be investigated if this is the case.
Seabirds
Populations of most seabird species in the Norwegian Sea are still declining, but there are indications of a positive trend for some populations and individual breeding colonies (Figure 3.10). Both kittiwake and fulmar, which are pelagic surface-feeding species, are still declining steeply, but numbers of gannets are rising. Of the pelagic diving species, Brünnich’s guillemot and puffin are declining, as is the common guillemot colony on Jan Mayen. There are signs of improvement at certain colonies of common guillemot and razorbill. As regards coastal species, great skua numbers are rising or stable, while populations of gulls and terns, shag and cormorant are stable or declining populations.
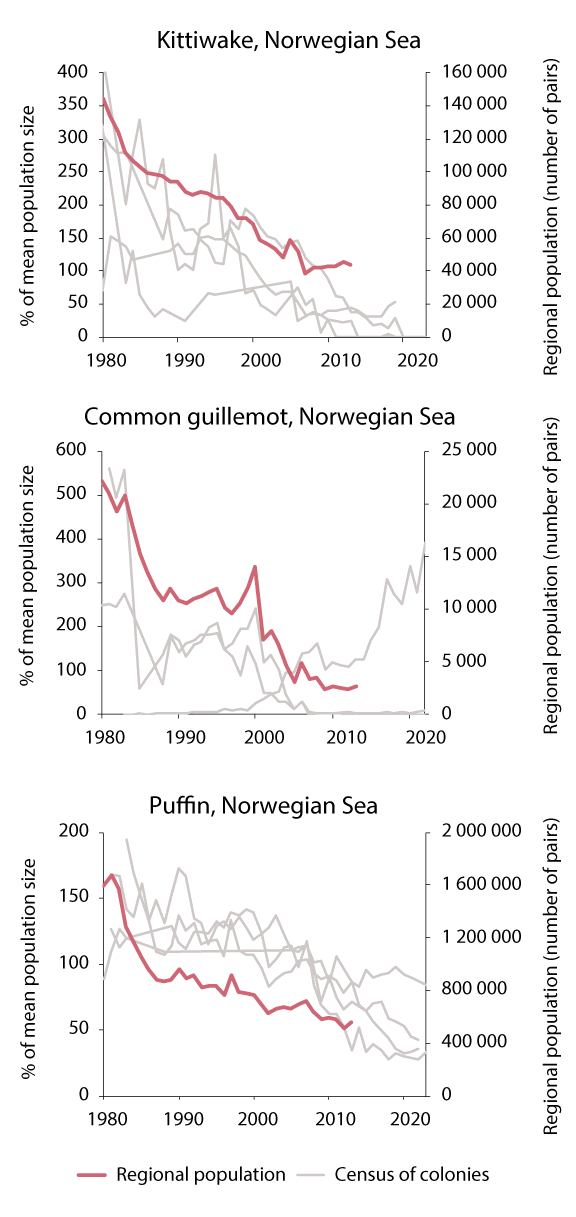
Figure 3.14 Seabird populations in the Norwegian Sea. Population trajectories for kittiwake, common guillemot and puffin. Bold red lines show the estimated population size, thin grey lines show figures from monitoring at specific colonies.
Sources: Regional population figures from NINA Report 1151 (2015), monitoring data from seapop.no
The breeding populations of common eider in the Norwegian Sea have declined greatly all along the coast from Møre og Romsdal county to Røst at the southern tip of the Lofoten Islands (Figure 3.15). Causes of the decline in seabird populations are discussed in Chapter 3.1.2.
Textbox 3.3 Common eider
Predation by mink, ravens, gulls and white-tailed eagles, which take eggs, chicks and adult birds, affects the common eider population. Historically, there was a tradition of eiderdown ‘farming’ in coastal areas from Central Norway and northwards. People provided sheltered nesting sites for eider ducks and harvested the eiderdown. This system has been abandoned, leaving the common eider with less protection. Predation by mammals and raptors probably puts considerable pressure on the common eider, but this has not been quantified. Disturbance by people often causes incubating eider ducks to leave the nest, leaving eggs and chicks exposed to predators.
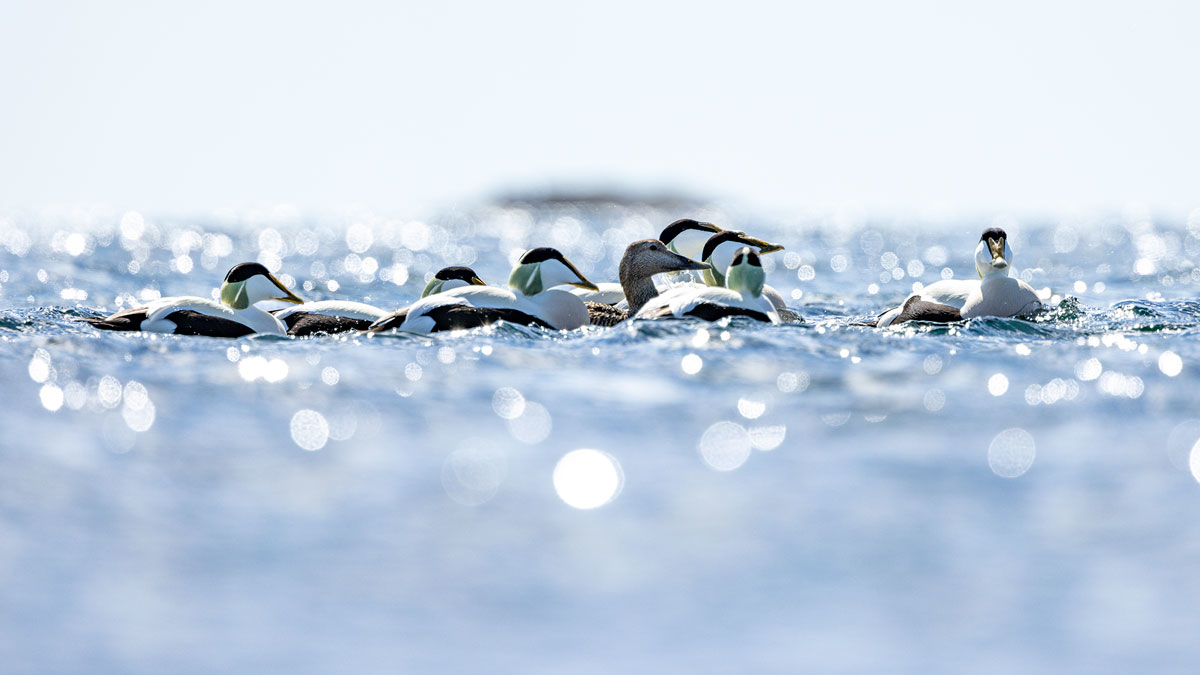
Figure 3.15 Common eider
Photo: Kim Abel/Naturarkivet
The population along the coast of Møre og Romsdal is now only 15 % of what it was in 1986. In the Trondheimsfjorden, the breeding population has dropped by 90 % since 1982, and in Leka municipality on the coast of Trøndelag, the population has declined by 80 % since 2001. In the southern half of Nordland county, the populations in the inner part of Ranfjorden and along the open coast have declined by 90 % and 75 % respectively since 2000. The breeding population on Røst has been reduced by about 75 % since 1988.
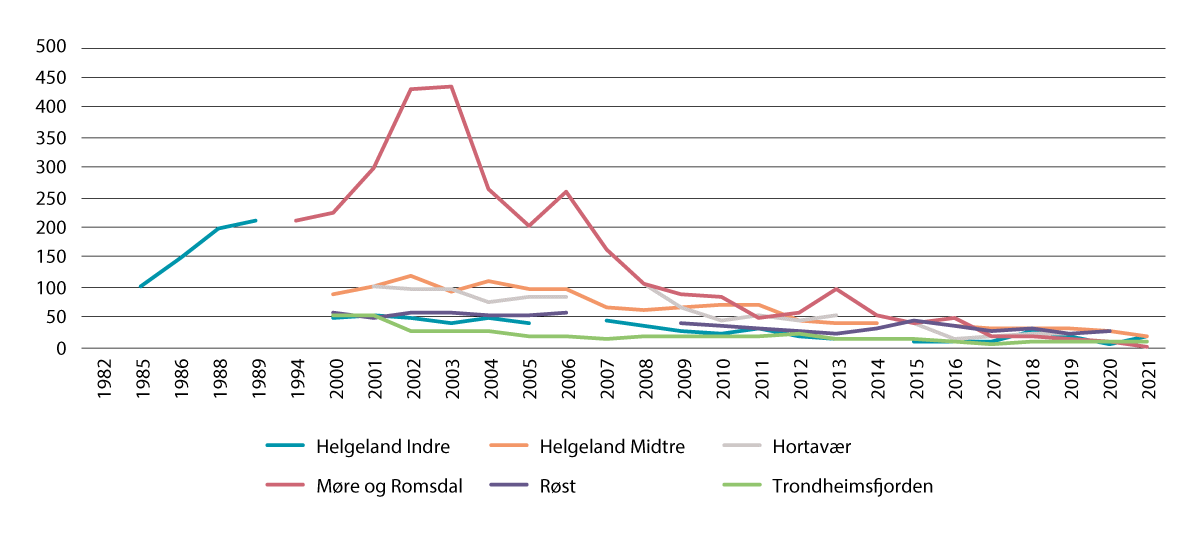
Figure 3.16 Population trajectories for common eider in Nordland county between the 1980s and 2021.
Source: Norwegian Institute for Nature Research/Miljøstatus
Marine mammals
The marine mammal fauna in the Atlantic part of the Norwegian Sea is dominated by whales. Some species, such as harbour porpoises and orcas, spend the whole year in the Norwegian Sea. Others, such as minke whales and other baleen whales, are present mainly in summer. The total minke whale population in the Northeast Atlantic has been stable, but in the past 10 –15 years there has been a certain decline in numbers in the Norwegian Sea and a corresponding increase in the Barents Sea. The minke whale is the only species that is harvested.
Population estimates for orca have varied considerably over time, but without any clear trend. This species feeds primarily on herring and mackerel in the Norwegian Sea, but a few groups also catch seals. Orcas that feed on seals contain very high levels of persistent, bioaccumulative and toxic substances, which may affect their health and reproductive capacity.
The harbour porpoise is relatively abundant, and is most widely distributed in coastal waters bordering on the Norwegian Sea management plan area. This species is particularly vulnerable to bycatches in gill nets, and rules have therefore been introduced requiring the use of pingers (acoustic deterrent devices) on gill nets in the Vestfjorden from 1 January to 30 April.
The hooded seal is one of the largest seal species in the North Atlantic, and also the one that dives deepest, to more than 1000 metres. The West Ice between Jan Mayen and Greenland is the only known whelping area for hooded seals in the Northeast Atlantic. This species is endangered. Until the 1980s, sealing was probably the most important driver for the hooded seal population, but the decline since then can probably be attributed to changes in reproduction rate and natural mortality. Both of these variables can be influenced by changes in food supplies. The hooded seal population has been protected since 2007, with the exception of a limited harvest for research purposes.
Coastal waters bordering the Norwegian Sea management plan area are important habitats for the relatively small Norwegian populations of grey and common seal. Regular monitoring since the late 1990s shows a general decline in pup production for grey seals and in the total population of common seals. Bycatches during gill netting for angler are believed to be one explanation for these trends.
Alien species
The alien species that have been registered in the Norwegian Sea are largely associated with coastal waters. The number of observations has risen in recent years, partly because of the continued spread of Pacific oysters (Crassostrea gigas) and pink salmon. By 2022, between 15 and 20 alien species had been registered. The comb jelly Mnemiopsis leidyi has spread rapidly from the Oslofjorden to the Trondheimsfjorden, and can dominate plankton communities locally in late summer and autumn. There are individual records of Pacific oyster from the southern part of the Møre og Romsdal coast, which indicates that the species may be spreading further northwards. The carpet sea squirt (Didemnum vexillum) has not yet been found in the Norwegian Sea, but it is probably only a matter of time before it spreads northwards.
Threatened species and habitat types
In all, 36 species in the Norwegian Sea, including algae, are listed as threatened (in one of the categories critically endangered, endangered or vulnerable). Conservation status is poorer for 13 species and better for 10 species than in the 2015 red list. Several species of annelid worms have been added to the list. Critically endangered species now include the black-headed gull, common guillemot, the skate Dipturus intermedius and shagreen ray (Leucoraja fullonica). Species listed as endangered include fish (golden redfish, blue ling, basking shark and European eel), marine mammals (hooded seal and bowhead whale), seabirds (puffin, fulmar and common tern), and the Baltic stonewort Chara baltica.
Four habitat types in the Norwegian Sea have been red-listed. Northerly sugar kelp forests are now classed as endangered because of intense grazing by sea urchins. The conservation status for coral reefs has improved from vulnerable to near-threatened. The other two habitat types are oarweed forests and mussel beds that are exposed to wave action, both of which are listed as vulnerable.
3.2.4 Pollution
Transport in the atmosphere and with ocean currents is the main source of inputs of hazardous substances and other pollutants to the Norwegian Sea. There are also inputs of pollutants via rivers, with runoff from land, and from activities in coastal waters and at sea. Oil and other naturally occurring substances are released with produced water and chemicals from petroleum activities in the Norwegian Sea, and there are also releases of oil and chemicals from shipping. There is a great deal of oil and gas activity in the Norwegian Sea, and releases of pollutants are relatively high, but because there are fewer fields in production, considerably lower than in the North Sea–Skagerrak management plan area. The industry is responsible for environmental monitoring programmes, which these generally show that releases of pollutants have only limited local impacts.
The volume of shipping in the Norwegian Sea is lower than in the North Sea and Skagerrak, but higher than in the Barents Sea–Lofoten area. Shipping in the Norwegian Sea is dominated by passenger transport. Given the expected increase in activity, operational releases of oil and chemicals will rise, as will the level of underwater noise, and the risk of the spread of alien species will increase.
Inputs of heavy metals from the atmosphere are low and declining. This is a result of reductions in emissions in Central and Eastern Europe. Atmospheric inputs of persistent organic pollutants (HCB and perfluoroalkyl substances (PFAS)) are stable or decreasing.
Estimates of annual inputs of nitrogen and phosphorus, and the heavy metals copper and lead to the coastline bordering the Norwegian Sea are based on data from monitoring of river systems, modelling results and data reported by industry, waste water treatment plants and fish farms. Inputs of lead have been declining since the late 1990s, and have levelled off at a low level after 2015. However, inputs of copper have risen in the past 10 years. Both direct releases of phosphorus and nitrogen and inputs via rivers have increased a great deal, and have almost doubled in the past 20 years (Figure 3.17). Rising inputs of phosphorus, nitrogen and copper are largely explained by an increase in discharges from the aquaculture industry. The use of copper for impregnation in the aquaculture industry has been somewhat reduced in recent years. Little is known about how nutrients are transported from coastal waters to the open sea. It is therefore unclear what proportion of nutrient inputs is transported from coastal waters into the management plan area.
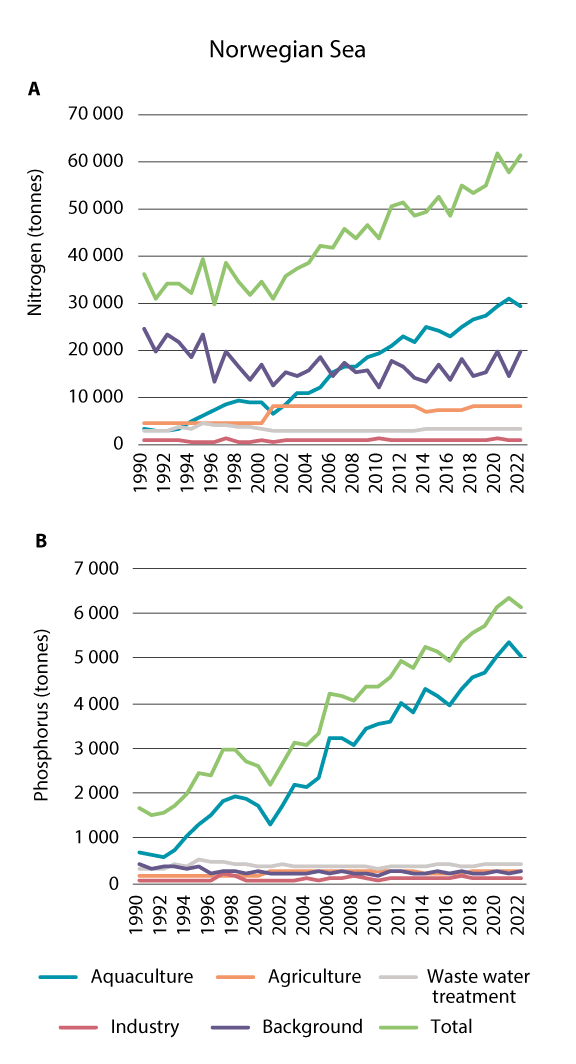
Figure 3.17 Inputs of nitrogen (a) and phosphorus (b) to the coastline bordering the Norwegian Sea management plan area in the period 1990-2022.
Source: NIVA report 7963-2024
In the Norwegian Sea management plan area, levels of persistent, bioaccumulative and toxic substances are monitored in selected species including mussels, shrimps, shag (eggs) and some commercial fish species. Seafood from the Norwegian Sea is generally safe to eat, since concentrations of contaminants are below the maximum permitted levels for food safety in edible parts of most species. There are a few exceptions. In an area around the outer Sklinnadjupet trench, fishing for halibut has been banned because high levels of mercury, dioxins and PCBs have been found in halibut caught in the area. Another exception is coastal cod. Levels of persistent, bioaccumulative and toxic substances in cod fillets are well below the maximum permitted levels for food safety. However, concentrations of such substances in the liver of coastal cod are often above the maximum permitted levels, and the Norwegian Food Safety Authority has issued a general warning to the population to avoid eating the liver of fish caught inside the baseline. High levels of cadmium have been measured in edible crabs caught along the coast from Saltfjorden (Nordland county) and northwards, and the Food Safety Authority has warned against catching crabs from this area. It is not known why unusually high levels of persistent, bioaccumulative and toxic substances, exceeding the maximum permitted levels, are found in a few cases and in specific areas in some seafood species caught in the Norwegian Sea.
Levels of persistent, bioaccumulative and toxic substances in sediments in the Norwegian Sea are generally very low.
Levels of radioactive substances from human activity are low in seawater along the coast and in the Norwegian Sea itself. As a result of stable or declining inputs and radioactive decay, these levels are stable or declining. Bladder wrack (Fucus vesiculosus) is a good indicator of trends in the level of technetium-99 (from the Sellafield plant) in marine organisms, since this seaweed accumulates technetium. Levels of technetium-99 in seawater are currently so low that they are generally below the detection limit, whereas levels in bladder wrack are low but quantifiable. The levels of caesium-137 (from the Chernobyl accident) measured in bladder wrack are also low. The dose from natural radioactive nuclides in seafood is greater than the dose from man-made substances, but the risk associated with natural radioactive substances in seafood is nevertheless low.
Fisheries-related waste, including lost fishing gear, is an important source of marine litter and plastic pollution in the Norwegian Sea. Other important sources are aquaculture and shipping. Most floating waste is of relatively local origin, but waste can also be transported from distant waters. Too little is known about marine litter and plastic pollution in this management plan area. Knowledge will be built up through national monitoring programmes for microplastics and macroplastics and more intensive monitoring of beach litter under OSPAR.
Underwater noise, for example from shipping, seismic surveys and sonar, is another form of pollution in the Norwegian Sea, but little is known about levels and effects.
3.3 Ecosystem condition, drivers of change and impacts in the North Sea–Skagerrak management plan area
In the North Sea and Skagerrak, anthropogenic climate change is resulting in higher temperatures and ocean acidification, and also coastal water darkening (increased light attenuation), probably because of increasing runoff of organic material from land. The assessment of ecosystem condition concludes that climate change and other anthropogenic pressures, particularly the fisheries, are having substantial impacts on the ecosystem. Ecosystem changes that have been observed involve key groups of zooplankton, fish stocks, shrimps and benthic communities. New data show a dramatic decline in a number of seabird populations that breed along the coast of the Skagerrak and North Sea. This management plan area is generally more polluted than the other two.
For the North Sea–Skagerrak, it was concluded that the sectors that make the greatest contribution to cumulative impacts on ecosystems are the fisheries, transport, sewage and wastewater treatment, agriculture, oil and gas and land-based industry. Selective species extraction, litter and pollution, and abrasion and smothering are particularly important pressures.
3.3.1 Ecosystem condition in the North Sea–Skagerrak management plan area
The assessment of ecosystem condition (see Box 3.1) for the North Sea and Skagerrak includes the entire management plan area except for the seabed and water column at depths below 200 m in the Norwegian Trench, which are considered to make up a different ecosystem from shelf ecosystem in the shallower parts of this area.
The impacts of human activity on the ecosystem in the Norwegian sector of the North Sea–Skagerrak are substantial. There is little uncertainty about this conclusion, which is based on an overall assessment using results for all seven of the ecosystem characteristics that were considered (Figure 3.18). Climate change is having considerable impacts on abiotic parts of the ecosystem, particularly through rising temperatures. Together with other drivers, such as the fisheries, this has substantial effects on the rest of the ecosystem. There are major impacts on key fish stocks and other functionally important species, and on benthic habitats. There are also signs that human activity has caused changes in species diversity and ecological functions and in biomass distribution among trophic levels.
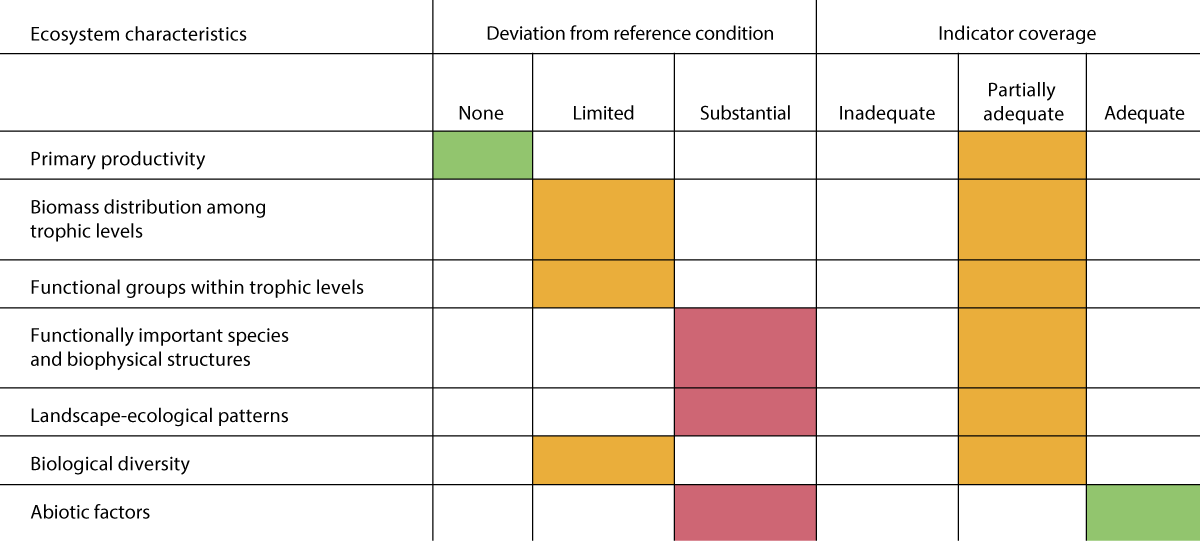
Figure 3.18 Summary of the assessment of ecosystem condition for each of the ecosystem characteristics for the North Sea-Skagerrak.
Source: Panel-based Assessment of Ecosystem Condition of the North Sea Shelf Ecosystem. Rapport fra havforskningen 2023-17.
The indicators for zooplankton account for a large proportion of the substantial deviation from the reference condition that was observed. Earlier overfishing is another important factor. Efforts to rebuild fish stocks after overfishing was stopped have been hampered by poor recruitment in the 2000s and 2010s. Zooplankton groups are important sources of food for fish larvae. For cod and herring, which have been thoroughly studied, poor recruitment has been clearly linked to the climate-driven changes in the zooplankton community. Another important aspect of human pressure on the ecosystem is the large proportion of the seabed and benthic communities that are affected by bottom trawling.
The ecological status of about half of the coastal water bodies in the North Sea–Skagerrak area is classified as good, and in some cases as high, according to the system used in the Water Management Regulations. The status of about 42 % of the water bodies is classed as poor and of 8 % as bad. The biological quality elements used to classify the ecological status of coastal water bodies are soft-bottom benthic invertebrates, eelgrass, macroalgae, and phytoplankton, and in addition there are physico-chemical quality elements.
3.3.2 Oceanic climate change: North Sea–Skagerrak management plan area
The ocean climate in the North Sea–Skagerrak area can be split into two main types, corresponding to the Atlantic deep water in the northern North Sea and the Norwegian Trench, and the North Sea water in continental shelf areas. The North Sea water is strongly influenced by local weather conditions, and there is considerable variability between seasons and years. The deep water, on the other hand, is strongly influenced by the Norwegian Atlantic current that flows in from the Norwegian Sea and southwards along the Norwegian Trench.
Towards the end of the 1980s, the temperature of both the surface water and the deep water in the North Sea rose by about 1 °C, and has remained at about this level since (Figure 3.19).
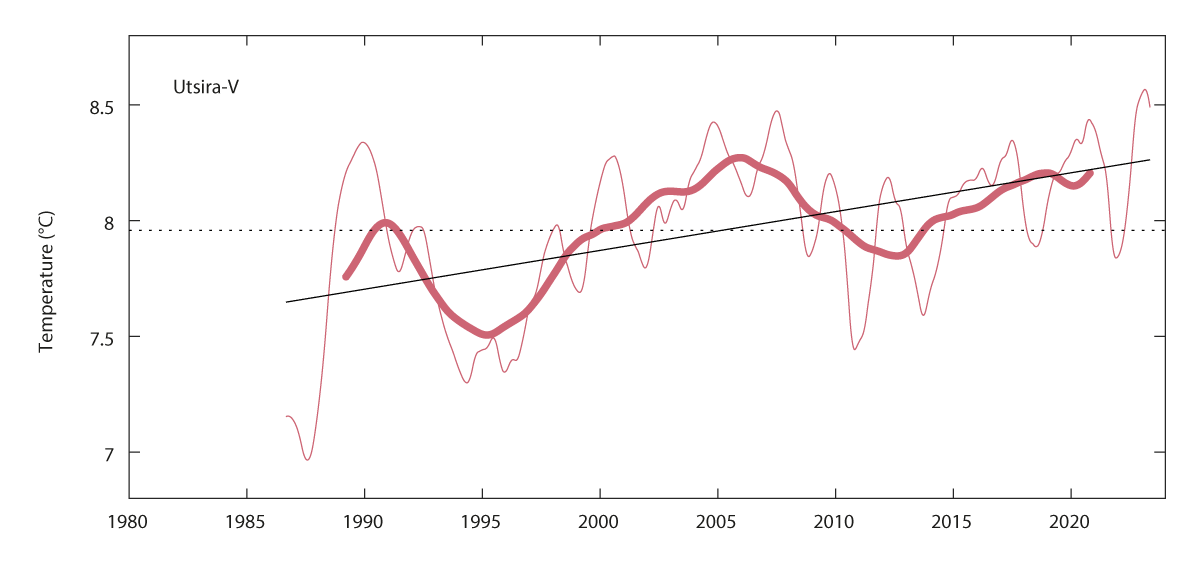
Figure 3.19 Temperature for the period 1980-2020 averaged within the depth interval 50-200 m, in the core of Atlantic water in the North Sea (Utsira-V section). The narrow red line shows annual means, the bold red line shows a smoothed curve using five-year means, and the black line shows the linear trend.
Source: Institute of Marine Research
Although only short time series are available and there is wide geographical and temporal variability in CO2 content and pH values in the North Sea and Skagerrak, signs of increasing ocean acidification can be seen (lower pH and lower aragonite saturation levels). Acidification is largely explained by increasing CO2 levels in seawater as a result of higher atmospheric emissions. Changes in the water masses or inputs of freshwater also add to ocean acidification.
The accumulation of particulate organic matter, particularly in deep-water basins where bacteria consume oxygen during degradation processes, can result in low oxygen levels at certain times of year. However, regular turnover and renewal of the bottom water improves the conditions. Since 1980, there has been a weak decline in oxygen levels in the Skagerrak bottom water (Norwegian Trench). Nevertheless, oxygen levels in the bottom water are considered to be satisfactory.
Observed effects of changes in ocean climate
The rising sea temperature is one factor behind the northward shift in the distribution of various zooplankton species, and the enhanced survival of more southerly zooplankton species. In the North Sea, there has been a shift in the copepod fauna from dominance of Calanus finmarchicus to dominance of C. helgolandicus. There has also been a decline in the total biomass of the copepod genera Pseudocalanus and Paracalanus. This has been related to a reduction in primary production, which in turn is linked to climate change. These changes have had impacts on many links in food chains.
Climate change may have an indirect effect on recruitment to fish stocks through changes in zooplankton communities. There is strong evidence that recruitment to both cod and herring stocks has declined substantially as a result of changes in zooplankton communities that result in poorer food supplies for the youngest life stages of fish. This also affects food supplies for seabirds, and is one of the causes of the decline in seabird populations in the North Sea and Skagerrak.
The coastal water has become markedly darker, probably because of increasing runoff of organic matter from land. This is linked to higher precipitation levels on land as a result of climate change. A reduction in the lower growth limit has been observed for several species of macroalgae in the Skagerrak, and this is partly attributed to light attenuation. In the North Sea, increased light attenuation has resulted in a delay in the spring algal bloom.
Textbox 3.4 Coastal water darkening
There has been an increase in runoff and inputs of dissolved organic matter from land to coastal waters in Norway in the past 30 years. This is a result of climate change and other anthropogenic drivers, and the trend is expected to continue in the future. Areas that are particularly vulnerable to changes in light penetration in water are fjords, the Skagerrak, the North Sea and the waters around Svalbard.
Marked darkening of the water has been observed in the North Sea and Skagerrak, probably because of increasing runoff of organic matter from land. Dissolved organic matter makes the water darker and less transparent. Changes in light penetration influence the depth of the euphotic zone (where sufficient light is available), and have impacts on all organisms that need light for photosynthesis and growth, such as phytoplankton, seaweed, kelp and eelgrass. Predators that hunt by sight are also affected by reduced light penetration. A reduction in the lower growth limit has been observed for several species of macroalgae (seaweed and kelp) in the Skagerrak, and this is partly attributed to light attenuation.
Water darkening has been found to delay the spring algal bloom in the North Sea, which has an important ecological function. A later spring bloom may reduce the food supplies available to the zooplankton, and in turn reduce the availability of food (quantities and/or timing) for juvenile fish, seabirds, etc. Thus, the effects of water darkening can spread throughout the food web.
3.3.3 Condition and trends for various ecosystem components in the North Sea and Skagerrak
Kelp forests
Sugar kelp forests are an important habitat type in Norway’s coastal waters, and are nursery areas and habitats for many marine species. In the Skagerrak and southwestern Norway, a number of factors have had substantial impacts on sugar kelp forests and eelgrass meadows. Since 2000, there has been a weak improvement in the condition of sugar kelp forests, but over the past 50 years there has been a decline in the distribution of this species in the southern half of Norway, particularly in the Skagerrak. This is probably a result of rising temperatures and nutrient levels, together with an increase in levels of particulate matter and humus, all of which have negative impacts on the sugar kelp growth and recruitment. Where sugar kelp has declined, areas have become dominated by filamentous algae. The greatest losses took place in the Skagerrak around 2000, but there has also been a considerable loss of sugar kelp in the North Sea. Southern sugar kelp forests are categorised as endangered on Norway’s red list. In the long term, there is a risk that the situation will deteriorate because a continued rise in temperature and in runoff from land will increase levels of nutrients and particulate matter in the sea.
The benthic fauna
There are no indicators for the benthic fauna in the North Sea and Skagerrak, and no regular monitoring except of shrimps (Pandalus borealis). The shrimp stock reached a historical low in 2012, then increased up to 2016, but was very low again in 2022. Its distribution has declined, and the density of shrimps in the Norwegian Trench off Rogaland and northwards is now very low. Recent surveys have shown that shrimps have also disappeared from fjords in Vestland county.
There is a high level of bottom trawling activity in the North Sea and Skagerrak, and the impacts can be seen across a large proportion of the seabed and benthic communities. These impacts may have consequences for ecological functions and the productivity of benthic communities.
Fish stocks
The important commercial stocks of mackerel, North Sea herring, Norway pout and sandeel are in good condition, but the coastal cod stocks are in poor condition. The stocks of North Sea saithe and North Sea cod are at low levels. Mackerel numbers in the North Sea have risen considerably in recent years. The mackerel stock is nevertheless still at a relatively low level, but is stable. Harvesting from the component of the mackerel stock that spawns in the North Sea is strictly limited, and it thought that this component of the stock is still in poor condition.
After 10 years of weak recruitment between 2000 and 2010, the sandeel stock in the central North Sea has now been strengthened. The stock on the Viking Bank is still very low. Sandeels are an important prey species. Low sandeel numbers have a strong influence on food availability for seabirds and minke whales.
After a period of gradual increase in the North Sea cod stock, the spawning stock has declined steeply in the past three years (Figure 3.20). Three substocks of North Sea cod have recently been identified (northwestern, Viking, and southern cod substocks). The northwestern substock is largest and in good condition. The Viking substock is smaller, but may increase as a result of strong recruitment. The southern substock is in poor condition and recruitment is very low.
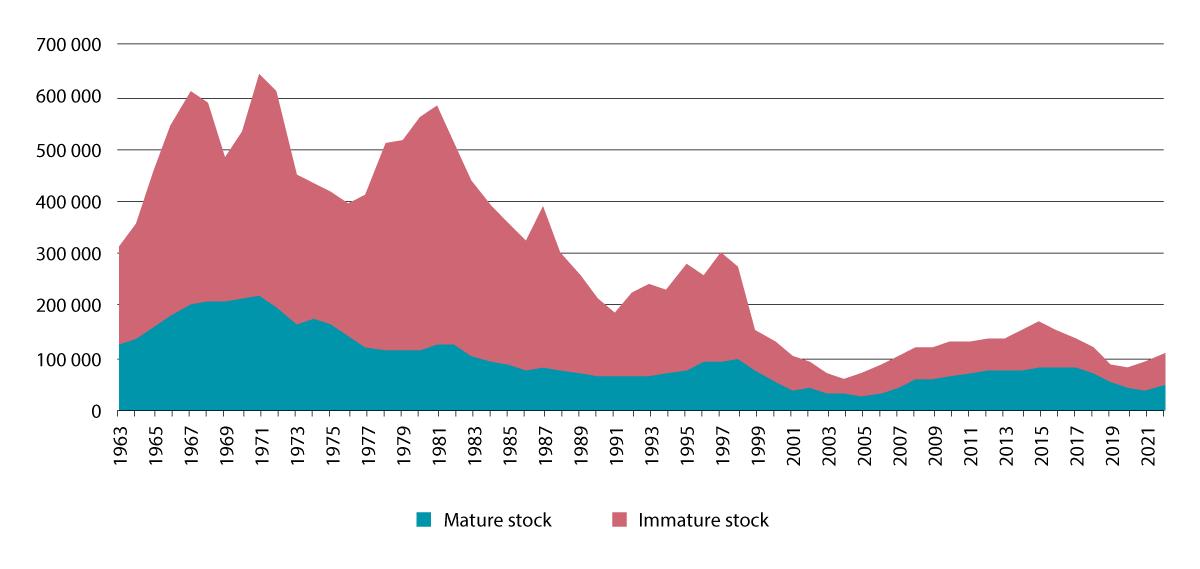
Figure 3.20 Stock trajectories for cod in the North Sea
Source: Institute of Marine Research
The haddock stock is now high as a result of limited harvesting and several strong year classes in recent years. However, the rise in water temperature is putting pressure on the stock. The North Sea saithe stock is declining and recruitment is weak, but the reasons for this are uncertain. There are signs that the stock may be stabilising at a low level.
The stock of North Sea herring is in good condition. The new year classes after 2001 have been weak, with the exception of the 2013 year class, but management of the herring stock is designed to take this into account.
The stocks of golden redfish and blue ling are at low levels (golden redfish is classed as endangered) and coastal cod stocks are in poor condition.
Seabirds
Populations of most seabird species in the North Sea–Skagerrak area are still declining steeply (Figure 3.21), but there are signs of a positive trend for certain populations and individual colonies. Populations of pelagic surface-feeding species are showing the steepest decline. The fulmar is disappearing as a breeding species in the management plan area. Colonies of the coastal species great black-backed, herring and lesser black-backed gull and common tern are still declining. The colonies of cormorant and shag are growing, but common eider populations are declining. Many of the negative changes in in seabird populations are linked to food shortages combined with climate change (see Chapter 3.1.2).
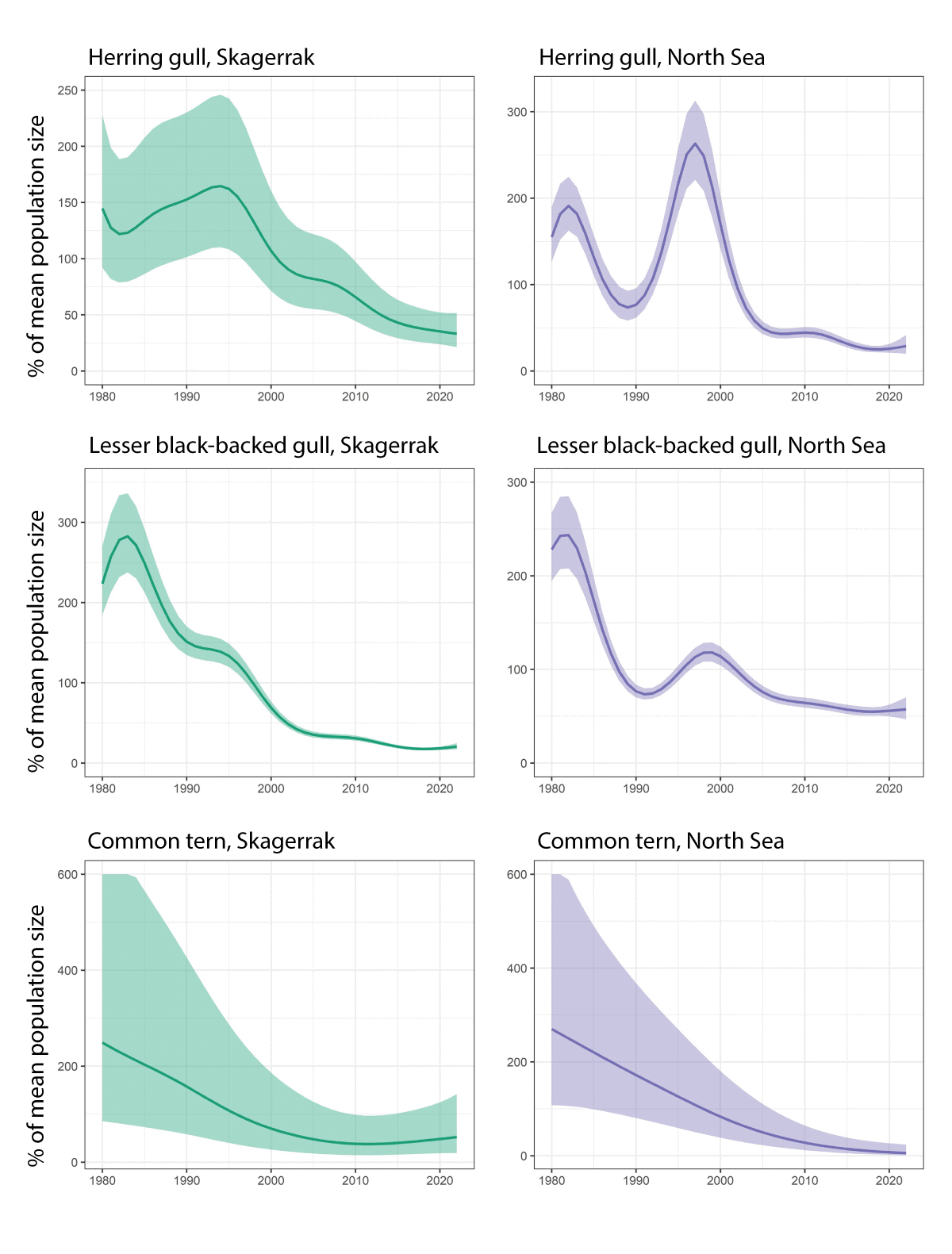
Figure 3.21 Population trajectories for herring gull, lesser black-backed gull and common tern in bird reserves in the North Sea-Skagerrak area. The figures show average trends with 95 % confidence intervals.
Source: Norwegian Institute of Nature Research
Marine mammals
The dominant marine mammal in the North Sea is the harbour porpoise, a small toothed whale with an estimated population of about 91 000 based on recent surveys. Porpoises are vulnerable to bycatches in the fisheries, but this does not appear to have resulted in population decline in the North Sea–Skagerrak area. Other toothed whales such as orcas and white-beaked dolphins are also observed regularly in the management plan area, but in small numbers. There are considerable numbers of minke whales in the North Sea in summer, but their abundance has varied widely according to surveys in the past 30 years.
There are two coastal seal species in the management plan area. The grey seal occurs from Rogaland and northwards. The estimated total population in the management plan area is around 200 animals. In 2022, the total population of common seal in the area was estimated at a minimum of 1689 animals, which is more than twice the estimate from the period 2016–2021. Seals are harvested as a renewable resource.
Alien species
There are more alien species in the North Sea–Skagerrak area than anywhere else in Norway’s marine and coastal waters. This is probably because of the greater volume of shipping and higher level of activity in this area, and also because the ocean currents in Norway’s southerly waters are more liable to spread alien species that have already become established elsewhere in Europe. Most alien species in the North Sea–Skagerrak area are associated with coastal waters. Examples include Pacific oysters, japweed (Sargassum muticum), the comb jelly Mnemiopsis leidyi and carpet sea squirt.
Pacific oysters can form very dense oyster reefs and alter coastal habitats. In some areas, the species can compete strongly with mussels and to some extent with European flat oysters. Pacific oysters have become established along the Skagerrak and North Sea coast of Norway as far north as Bergen. The species may still be in an early phase of establishment along the coast of Western Norway. It is dependent on fairly high summer temperatures for successful spawning, and is also sensitive to low winter temperatures.
The carpet sea squirt was found in the port of Stavanger in 2020. This species is spread by shipping through hull fouling and on other objects floating in the sea. It tolerates temperatures between -2 and 24°C and can therefore thrive along most of the Norwegian coast. It appears to be spreading rapidly along the coast of Western Norway. It can overgrow and displace most other naturally occurring filter feeders (for example mussels and oysters). It can also have an impact on other habitat types in shallow waters such as Laminaria hyperborea kelp forests, eelgrass meadows and maerl beds.
Threatened species and habitat types
The number of threatened species in the North Sea–Skagerrak management plan area has continued to increase. In all, 33 species in the area are listed as threatened (in one of the categories critically endangered, endangered or vulnerable) in Norway’s 2021 red list. Conservation status is poorer for 16 species and better for four species than in the 2015 red list. Several species of annelid worms have been added to the list. The common guillemot and the skate Dipturus intermedius are still listed as critically endangered, and the shagreen ray and black-headed gull are now also classed as critically endangered. Species listed as endangered include fish (blue ling, golden redfish, basking shark, European eel) seabirds (kittiwake, common tern, puffin and fulmar) and two species of annelid worms. The common eider population has continued to decline, and the species has now been classed as endangered.
Three deep- and shallow-water marine habitat types are classed as threatened. Bamboo coral gardens are now considered to be endangered both in the North Sea and in the Skagerrak. Bottom trawling (for shrimps) is widespread within the distribution area of bamboo coral in the North Sea, and is liable to damage this habitat type. Sugar kelp forests are also endangered as a result of pollution and climate change. The situation for mussel beds that are exposed to wave action has improved. The conservation status of coral reefs has improved from vulnerable to near-threatened.
3.3.4 Pollution
The North Sea-Skagerrak area is generally more polluted than the other two management plan areas. Inputs of persistent, bioaccumulative and toxic substances and other pollutants to this area take place with ocean currents and in the atmosphere, with rivers, in runoff from land, in discharges from land-based industry, and may also originate from petroleum activities, the fisheries, aquaculture and shipping.
There is a higher level of activity in the petroleum industry and maritime transport in the North Sea and Skagerrak than in the other management plan areas. The largest source of oil discharges to the North Sea is the continual release of produced water from offshore oil and gas extraction. There has been a weak reduction in releases of oil with produced water in the North Sea since 2015. Monitoring has identified impacts of oil pollution on haddock caught near petroleum installations in the North Sea, in the form of DNA damage as a result of exposure to PAHs (polycyclic aromatic hydrocarbons). It is uncertain how much of this can be attributed to earlier discharges of drill cuttings contaminated with oil-based drilling fluids, and how much to operational discharges in connection with current activities. Samples of other fish species have not shown similar effects. We lack recent modelling results for operational discharges of oil from shipping.
Across most of the North Sea, inputs via the atmosphere appear to be most important source for most of the persistent, bioaccumulative and toxic substances that have been investigated: mercury, most other heavy metals, PAHs and PCBs. In the Skagerrak, inputs from rivers and areas near the coast are most important for most heavy metals and PCBs. Atmospheric inputs of most heavy metals and other persistent, bioaccumulative and toxic substances that are monitored have declined considerably since measurements started during the 1990s. Inputs have levelled off at low levels in recent years. The reduction in inputs can be explained by large emission reductions in other European countries.
Levels of most contaminants in seafood species from the management plan area are below the maximum permitted levels for food safety. In certain cases, for example shrimps, the levels of contaminants are higher than the Environmental Quality Standards (EQS), and could potentially have an impact on animals at the top trophic levels, such as seabirds and marine mammals. We lack indicators for the levels and effects of hazardous substances in vulnerable top predators such as seabirds and marine mammals in the North Sea.
Levels of man-made radioactive substances in the North Sea and Skagerrak are low, but somewhat higher than in the Norwegian Sea and Barents Sea–Lofoten area. This is explained by the outflow of water from the Baltic Sea, which contains higher concentrations of caesium-137 from the 1986 Chernobyl accident. The oil and gas industry releases naturally occurring radioactive substances in produced water, but on most fields the levels of radioactivity involved are low. The largest releases are in the North Sea. No negative environmental impacts have been identified at the levels of radioactive pollution found in Norway’s marine and coastal waters.
Patterns of inputs of nutrients differ between the North Sea and the Skagerrak. Inputs of phosphorus and nitrogen and of organic matter to the Skagerrak coast vary from year to year but with no clear trend over time. Inputs of copper have declined. In contrast, inputs of phosphorus to the North Sea coast show a large increase throughout the whole measurement period.
Inputs of nitrogen show a marked rise from 2000 to 2015, but have since declined somewhat. The rise in inputs of nutrients (Figure 3.22) and copper to the North Sea is to a large extent linked to releases from fish farming. The use of copper for impregnation in the aquaculture industry has been somewhat reduced in recent years. Little is known about how nutrients are transported from coastal waters to the open sea.
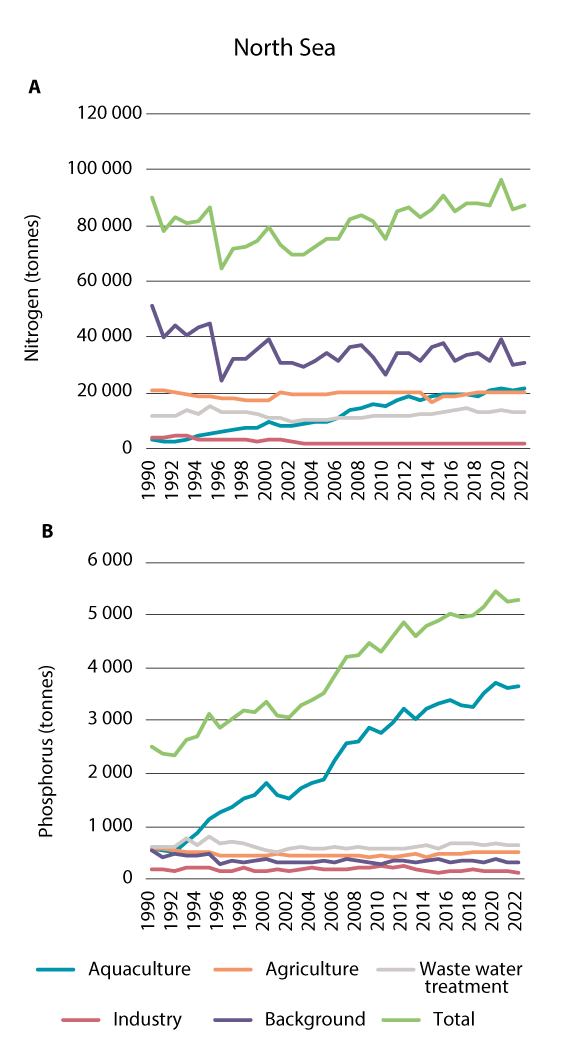
Figure 3.22 Inputs of nitrogen (a) and phosphorus (b) to the coastline bordering the North Sea-Skagerrak management plan area in the period 1990-2022.
Source: NIVA report 7963-2024
Runoff of nutrients from land and transport with ocean currents can carry nutrients from coastal waters to the open sea. The concentration of nutrients is measured in the water column in the Skagerrak. Over the past 20 years, the concentration of nitrogen in open waters in the Skagerrak has declined.
There have been improvements in nutrient levels over the years. There is no eutrophication in open waters in the Skagerrak, but certain areas close to the coast do show signs of eutrophication, for example the Oslofjord.
There are many sources of underwater noise in the North Sea and Skagerrak. Underwater noise from shipping appears to be particularly intense in the Norwegian sector of the North Sea. The level of seismic activity is also high here. Underwater noise can alter the behaviour of a variety of marine organisms including marine mammals, seabirds and fish, but currently little is known about possible effects at population and ecosystem level.
The largest source of marine litter and plastic pollution in the North Sea and Skagerrak is consumer waste. Sea-based sources are also important, especially the fisheries. In the North Sea, fulmars are used as an indicator species for plastic pollution. The target is for the percentage of fulmars from the North Sea with more than 0.1 g plastic in the stomach to be below 10 %, but this has not been achieved. The percentage of fulmars with more plastic in their stomachs is considerably higher, and has been fairly constant at around 50 %. Monitoring of beach litter at Lista and Ytre Hvaler has shown no reduction in quantities. Too little is known about trends for marine litter and plastic pollution in this management plan area. Knowledge will be built up through national monitoring programmes for microplastics and macroplastics and more intensive monitoring of beach litter under OSPAR.
Ammunition dumped at sea
Several hundred thousand tonnes of ammunition and chemical weapons have been dumped in Norway, most of it at sea. However, much of this was never registered, and the real quantities are therefore unknown. Most of the dumped ammunition is from the Second World War. In the post-war years, entire ships carrying German chemical weapons were sunk in the Skagerrak, with the permission of the Norwegian authorities. At the time, this was regarded as a simple and low-cost way of dealing with the problem.
Ammunition contains explosives, heavy metals, and a variety of hazardous substances. The Norwegian Defence Research Establishment is mapping and carrying out environmental monitoring of dumping sites. Pollutants have started to leak from dumped ammunition, but little is known about the environmental impacts. The Defence Research Establishment has found that heavy metals, explosives and other substances are leaking from several dumping sites in Norwegian waters (in the Skagerrak, near Bergen and in Nordland and Troms counties). Investigations in the other four areas in the list have revealed residues of explosives in fish and shellfish near all the sites investigated, while residues of chemical warfare agents were found in fish and shellfish near the site investigated in the Skagerrak. Dumped ammunition poses a risk to the fishing industry and others using areas near the dumping sites. Explosives in ammunition that was dumped at sea or buried on land are often functional for long periods after they were produced, and may in some cases become more sensitive to disturbance over time. In the worst case, ammunition may self-detonate where it was dumped or if it is moved.
3.4 Analyses of future climate change in Norwegian waters
In its Sixth Assessment Report (2021–2023), the IPCC concludes that climate change is expected to intensify in all regions of the world, and that the global temperature rise will exceed 1.5°C in the next 20 years at current emission levels. The IPCC’s findings show that it is very likely that ocean acidification will continue to increase for several decades if global CO2 emissions go on rising. This will have major negative impacts on marine ecosystems. Knowledge about how climate change is likely to affect Norway’s coastal and marine waters has been considerably improved. In the previous white paper on the management plan areas, it was decided to conduct a risk analysis of direct and indirect effects of climate change on marine ecosystems under different emission scenarios, and this work has now been done. The Institute of Marine Research modelled changes in Norway’s ocean areas for three global emission scenarios, SSP1-2.6 (low), SSP2-4.5 (intermediate) and SSP5-8.5 (very high). Using the same emission scenarios, the Norwegian Institute of Water Research assessed how climate change will affect the physical and biological environment in Norwegian coastal waters. This section presents the main points from these two reports, supplemented with findings from the assessments of ecosystem condition.
3.4.1 Norway’s ocean areas – future climate change and impacts
Seawater temperatures will rise, both at the surface and in deeper water layers. Projections for surface water temperatures from 2015 to 2100 show only small expected changes under the low emission scenario, whereas very large changes are expected under the very high emission scenario towards the end of this century (Figure 3.23). Under this scenario, it is also expected that the sea ice will almost completely disappear by 2100. With rising temperatures, the oxygen content of the seawater will also decline, particularly under the very high emission scenario, and the greatest reduction will be in the northernmost parts of the Barents Sea. A reduction in pH is expected under both the intermediate and the very high emission scenario. The decline in pH is expected to be largest in the Arctic water in the easternmost parts of the Barents Sea.
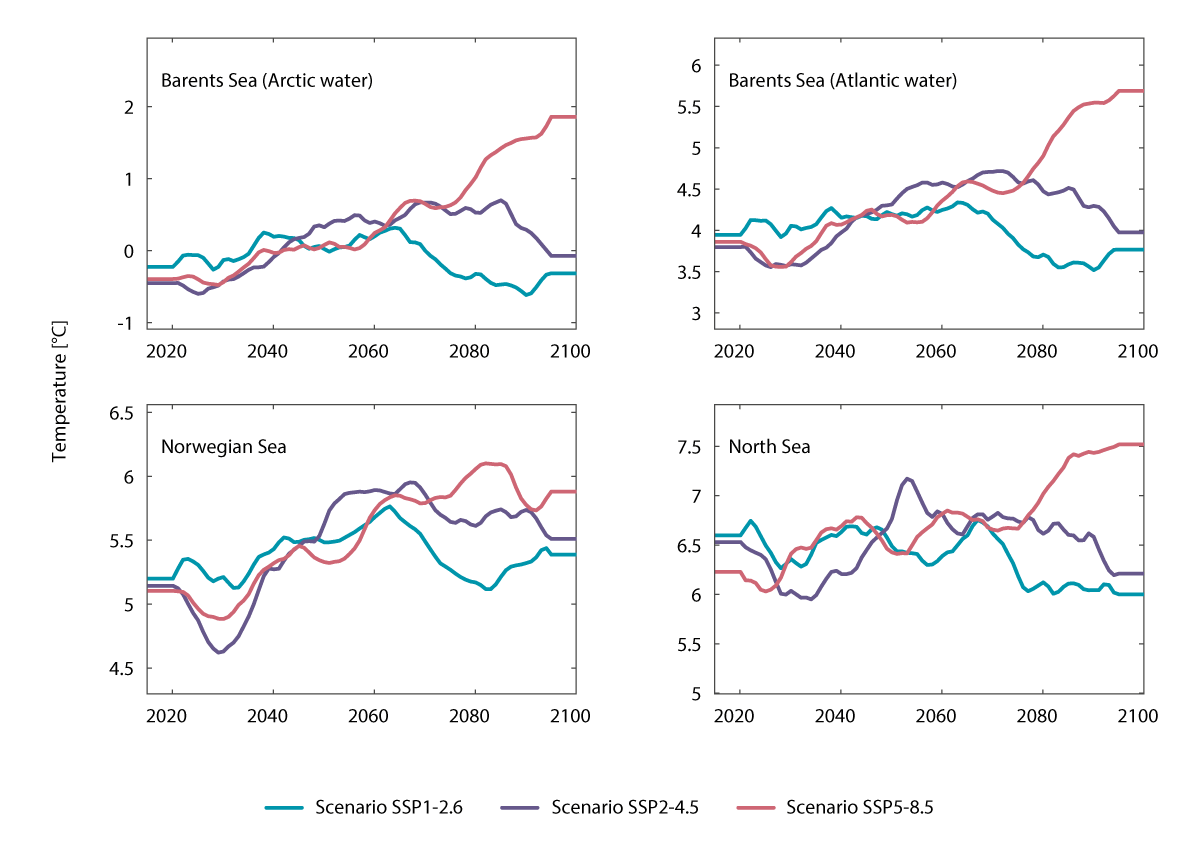
Figure 3.23 Projected surface water temperatures (°C) in March for the period 2015-2100 in ocean basins and neighbouring coastal waters. The colours of the time series indicate the emission scenarios used: SSP1-2.6 (blue), SSP2-4.5 (purple) and SSP5-8.5 (red). The low (SSP1) scenario represents a green pathway and sustainable growth. The intermediate scenario (SSP-2) represents a pathway following historical trends. The very high scenario (SSP5) represents a world of rapid and unlimited growth in production and energy use.
Source: Sandø A. B. et al (2022) Risikoanalyse for de norske havområdene om direkte og indirekte virkninger av klimaendringer på marine økosystemer under ulike utslippsscenarier [Risk analysis for Norway's ocean areas of direct and indirect effects of climate change on marine ecosystems and other relevant factors under different emission scenarios] Rapport fra havforskningen 2022-4
Primary production is expected to increase in northern parts of the Barents Sea with the retreat of the sea ice. This effect is particularly marked under the very high emission scenario. In the North Sea, primary production is expected to decrease, especially under the very high emission scenario. This is reflected in projected changes in secondary production, which is expected to increase in the Barents Sea and the Norwegian Sea, but decrease in the North Sea.
Changes in temperature, ice extent, oxygen content, pH, primary and secondary production, and knowledge about how sensitive different populations are to changes in these variables, were used as a basis for assessing the overall effects on 13 populations of selected key species of plankton, fish and shellfish.
It seems likely that the North Sea population of European hake, a temperate species, will benefit from a warmer ocean climate in the North Sea, while there will probably be negative impacts on other populations in the North Sea, for example North Sea herring, North Sea cod and the copepod Calanus finmarchicus. This applies especially to the North Sea cod population, since both direct effects of temperature on cod and indirect effects through changes in zooplankton production and species composition are important factors. C. finmarchicus provides an important link between primary production and fish and other organisms at higher trophic levels. It therefore plays a key role in Norwegian marine ecosystems. The projected decrease in secondary production is due to the expected drop in primary production.
Of the populations in the Norwegian Sea, European hake, Northeast Atlantic mackerel, Norwegian spring-spawning herring and C. finmarchicus will all benefit from higher temperatures. This conclusion is based on expansion of their distribution as a result of rising sea temperatures, and higher secondary production (food availability).
In the Barents Sea, a large increase in the population of Northeast Arctic cod is expected. This is explained by the loss of sea ice as temperatures rise, which will result in higher primary and secondary production in areas where the ice melts. Thus, rising temperatures will result in more abundant food supplies for Northeast Arctic cod and other species such as C. finmarchicus. However, the cod stock is currently declining despite warming sea temperatures. This is linked to weak recruitment, the cause of which is unknown. Under the very high emission scenario, the disappearance of the sea ice is very likely to have negative impacts on populations of species that are more closely associated with the marginal ice zone, such as capelin and Arctic cod. This effect may to some extent be counteracted by higher primary and secondary production. Because of this, the overall impact of climate change on capelin is expected to be positive. In contrast, climate change may have serious negative impacts on polar cod, since this species spawns under the sea ice and is closely associated with the marginal ice zone.
3.4.2 Norway’s coastal waters – future climate change and impacts
For Norwegian coastal waters, projected climate change during the 21st century will result in considerable warming and acidification of the seawater. The higher the level of greenhouse gas emissions are, the greater the changes are projected to be. Depending on latitude, the average surface water temperature will rise from the current level of 6.5–9 °C to 9–11°C under the intermediate emission scenario. As the water warms, it can hold less dissolved oxygen. Dissolved oxygen in the water column shows a declining trend up to 2100, but oxygen availability is not expected to become a limiting factor in Norwegian waters. The largest changes in temperature and oxygen content are expected to occur along the coast of North Norway. Acidification is expected to increase along the entire coastline, particularly under the very high emission scenario, with the largest reduction in pH in the southern half of the country. Salinity is expected to decline along the coast as a result of higher precipitation on land and an increase in runoff from rivers. The lowest salinity values for the whole Norwegian coast are measured in the surface waters of the Skagerrak, and this is also where the largest reductions in salinity are expected, especially under the very high emission scenario. An increase in runoff will also cause darkening of the water, particularly in the Norwegian coastal current, and this may have an impact on productivity in the areas affected.
Projected impacts on coastal species
An assessment was made of the impacts of projected climate change in Norway’s coastal waters on the quality and extent of the habitat used by five species – Atlantic salmon, coastal cod, the kelp Laminaria hyperborea, green sea urchin (Strongylocentrotus droebachiensis) and red king crab. These species play an important role in the coastal ecosystem and also for the regional and national economy, historical and cultural traditions and food security.
Changes in physical conditions along the Norwegian coast will result in changes in the distribution of many marine species, including the five selected for this analysis. The estimates for red king crab habitat indicate that the species will be mainly confined to North Norway, but even there, habitat quality will decline up to 2100 under all three scenarios. The estimates for coastal cod suggest that it will be able to find suitable habitat all along the Norwegian coast and through the entire period up to 2100. The best conditions will be in North Norway, where physical conditions will continue to be within the species’ tolerance limits in future, while temperatures in other parts of the country will gradually become too high. For Atlantic salmon, rising seawater temperatures will result in declining habitat quality in coastal areas, but coastal waters will continue to provide suitable habitat for the species under all three emission scenarios. Given its temperature preference, the distribution of L. hyperborea is expected to remain stable in southerly coastal waters and expand somewhat further north in the coming decades. However, the temperature will rise more steeply towards the end of the century under the intermediate and very high emission scenarios. The result is expected to be a decline in distribution or local extinction in the southern half of the country, respectively. The green sea urchin is generally resilient to climate change, and the estimates indicate that its distribution will be stable under the low and intermediate emission scenarios. Under the very high emission scenario, a steep decline ending in local extinction is expected along the whole of the Norwegian coast, mainly as a result of higher temperatures and a sharp drop in pH.
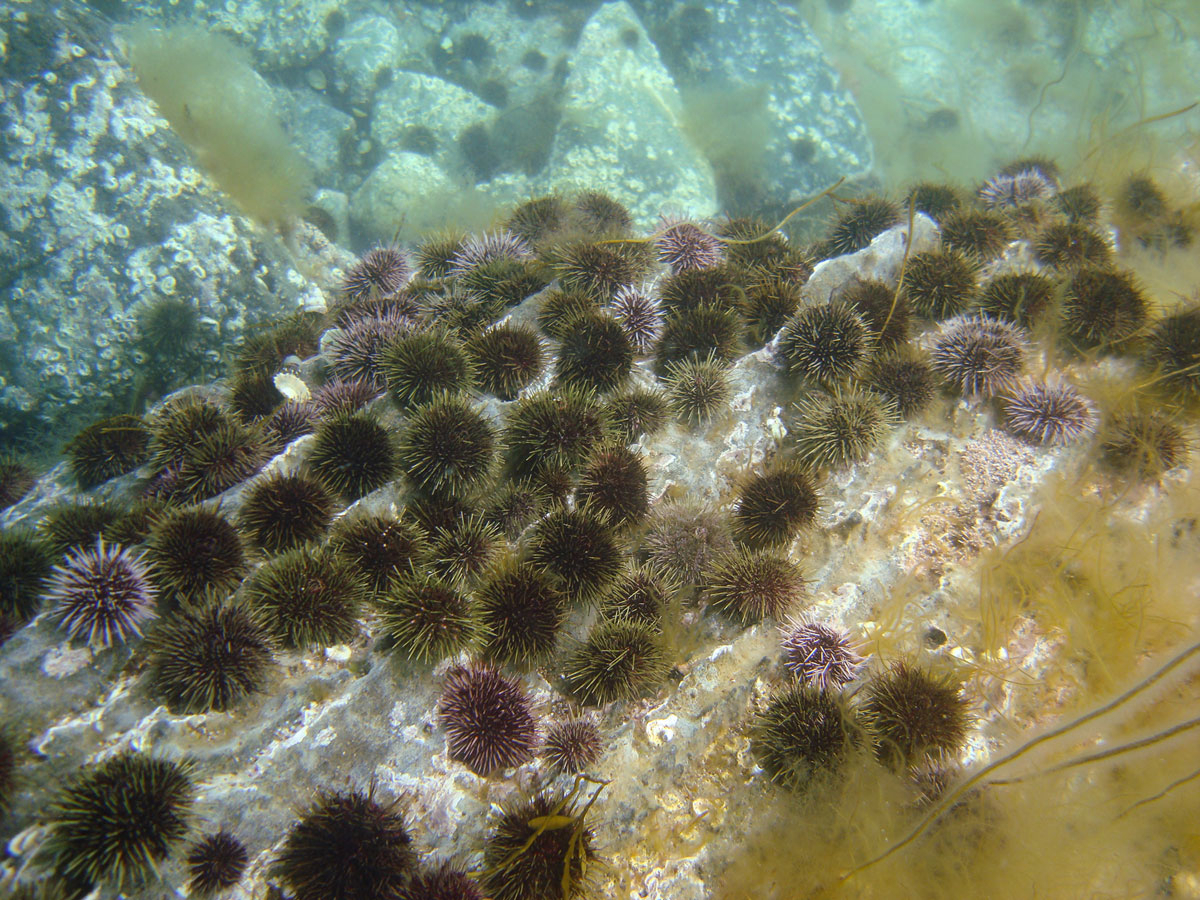
Figure 3.24 Green sea urchins in the Vega archipelago. Large areas of kelp forest along the coast of the Norwegian Sea have been overgrazed by sea urchins
Photo: Eli Rinde/ Norwegian Institute for Water Research
3.4.3 Marine heatwaves
As a consequence of global warming, both the frequency and the duration of marine heatwaves have increased over time in the world’s oceans, most rapidly in recent decades. They are expected to become even more frequent and more intense in the future. A marine heatwave can be defined as a discrete, prolonged (more than five days) anomalously warm water event. Marine heatwaves are described in terms of their duration, intensity, frequency and spatial extent. Heatwaves may be triggered by both large-scale and regional atmospheric and oceanic processes.
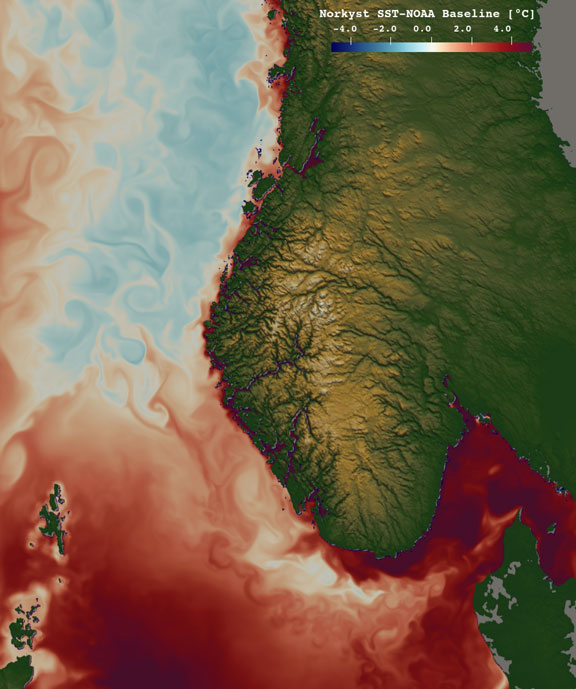
Figure 3.25 Marine heatwave in the North Sea, showing the sea surface temperature anomaly on 18 June 2023. This was during an intense marine heatwave in the North Atlantic and North Sea, with temperatures up to 4-5 °C higher than expected on the basis of the long-term mean for the period 1991-2020.
Data source: Norwegian Meteorological Institute, Norkyst_v3 model for coastal forecasting and NOAA Optimum Interpolation SST V2. Figure: Marta Trodahl, Norwegian Meteorological Institute
Knowledge about the scale and impacts of marine heatwaves is limited, but there is growing evidence of major biological impacts in many parts of the world’s oceans. The effects of marine heatwaves are linked to the heat tolerance of marine species and how mobile they are. Observed impacts of marine heatwaves in the Pacific, Atlantic and Indian Oceans include coral bleaching, toxic algal blooms, loss of kelp forests and seagrass meadows, and declining fish stocks or shifts in their distribution. Marine heatwaves are probably a greater threat to marine ecosystems than the gradual temperature rise associated with global warming. There are few studies of the possible effects of marine heatwaves on marine ecosystems in northern waters. Knowledge of possible impacts is based on studies from other regions with comparable ecosystems.
A literature review commissioned by the Norwegian Environment Agency contains a description of what is known about marine heatwaves in Nordic waters in the period 1982–2020. In waters north of 60 oN, which on both the Barents Sea and the Norwegian Sea, the intensity, duration, frequency and spatial extent of marine heatwaves have all increased during this period. In this region, the increases are correlated with rising air temperatures at the sea surface and a decline in sea ice extent. Marine heatwaves in Arctic waters are just as intense as or even more intense than those in other waters. There is a stronger increase in their frequency than in other waters.
The spatial extent of marine heatwaves in the Barents Sea varies widely. In the waters around Svalbard, there have been 2–3 marine heatwaves per year since 1982, while in the rest of the Barents Sea they occur 1–2 times a year. For the Barents Sea as a whole, the frequency of marine heatwaves has risen by 62 % over this period, and particularly strongly in the last decade. Since 1982, there have been 1–2 marine heatwaves per year in the Norwegian Sea. This is believed to be 2–4 times the frequency in the pre-industrial period.
The frequency of marine heatwaves is rising in the North Sea and Skagerrak as well. Sugar kelp is the dominant kelp species along the North Sea and Skagerrak coast of Norway. The decline of sugar kelp forests has been explained by a combination of eutrophication, a rise in the content of particulate matter in seawater, sediment deposition and higher water temperatures. New studies have shown that marine heatwaves may also be a cause of sugar kelp decline. Sea temperatures during marine heatwaves have regularly exceeded the lethal limit for sugar kelp.
An indirect positive effect of higher sea temperatures combined with marine heatwaves is that recruitment of sea urchins, which overgraze kelp forests, is declining in Norway’s southerly waters. The sea urchins do not tolerate such high water temperatures, and the main distribution area is shifting northwards. This has resulted in less overgrazing along the North Sea and Norwegian Sea coasts.
Using analyses of historical data for seawater temperatures combined with more intensive monitoring, particularly of species that are sensitive to temperature, it will be possible to build up more knowledge about marine heatwaves in Norwegian waters.
3.5 Knowledge building and knowledge needs
Knowledge-based management of Norwegian waters must be based on adequate knowledge about ecosystem condition, drivers of change and impacts. This is built up through mapping, monitoring and research. A considerable amount of knowledge has been built up since the previous white paper on Norway’s ocean management plans was published, especially about the particularly valuable and vulnerable areas. We have also learned more about the effects of climate change, the spread of microplastics and cumulative impacts. However, further work needs to be done to continue knowledge development, in particular about ecosystem condition, changes, the impacts of drivers, causal relationships and cumulative impacts. Important knowledge needs relating to integrated ocean management are also discussed in the white paper on a long-term plan for research and higher education 2023–2032 (Meld. St. 5 (2022–2023)) under the thematic priority oceans and coastal waters.
Biodiversity and ecosystems
Mapping and monitoring are needed in areas where knowledge is currently limited, for example open waters in the Barents Sea and the Norwegian Sea. In areas that are already better known, there should be long-term monitoring of species and habitats and the physical environment. Monitoring programmes need to include organisms at all trophic levels and in all functional groups in representative marine ecosystems.
More knowledge is needed about how climate change, pollution and environmental conditions influence population, species and ecosystem trends, and what can be done to repair damage that has been done to species and habitat types.
In addition, the distribution of endangered marine habitat types needs to be mapped. We also need to learn more about ecological processes such as interactions between species and trophic levels. Moreover, we need to build up knowledge about factors that affect the vulnerability vulnerable of species to habitat change. More knowledge is needed about the causes of changes in seabird populations.
There is a need for more systematic monitoring of the spread of alien species and their impacts on native species and habitat types, particularly near the coast. In addition, we need to learn more about pathways of introduction, how to avoid the spread of alien species, and how to contain and control alien species that have already become established.
Climate change and ocean acidification
Since the previous white paper on the management plans was published, a great deal of new knowledge has been obtained about climate change and its effects in Norway’s ocean areas. Nevertheless, there are still knowledge gaps as regards current and future effects of climate change on natural carbon stores, levels of hazardous substances in ecosystems and their effects, and the spread of alien species. Marine habitat types and species that are vulnerable to and/or are being adversely affected by climate change and ocean acidification should be mapped and monitored.
Pollution and litter
Much more information is still needed to give a complete picture of the levels of hazardous substances in the management plan areas. The monitoring programmes only include a limited selection of substances, and new chemicals are constantly being registered in these areas. More knowledge is needed both about the levels of ‘new’ substances, including micro- and nanoplastics, and about their implications for seafood safety and human health. Our knowledge about concentrations of persistent, bioaccumulative and toxic substances in organisms at the lowest trophic levels is also limited and should be further developed. The same applies to knowledge about the effects on fish, seabirds and marine mammals.
Marine organisms are exposed to many different substances simultaneously, and more knowledge is needed about critical levels of exposure to persistent, bioaccumulative and toxic substances and about the cocktail effects of releases of multiple pollutants. In the North Sea and Skagerrak, regular monitoring of levels of metals in sediments is needed. The Environmental Specimen Bank Norway is one important tool in national and international efforts to deal with hazardous substances. Environmental samples stored here provide an archive of the past for future use. More knowledge is also needed about levels of plastic pollution and about its spread and impacts, particularly as regards micro- and nanoplastics. It is important to use suitable indicators for monitoring based on countries’ national monitoring programmes, and to implement and harmonise programmes with regional and international initiatives. More knowledge is needed about levels of micro- and nanoplastics, and chemicals associated with them, in the environment and in seafood. We also need a better understanding of the implications for seafood safety and human health. Our understanding of the impacts of underwater noise on ecosystems is also inadequate. In addition, we need to build up knowledge of indicators for underwater noise (pressure and ecosystem effects), vulnerability to noise at population level and threshold levels for behavioural change, for example in fish.
Radioactivity in seawater is already being monitored, but more knowledge is needed on uptake, accumulation and possible impacts of radioactive pollution of the marine environment. We also know too little about various aspects of operational releases from the offshore oil and gas industry. For example, little is known about the effects of complex mixtures of chemicals, or about the long-term effects of exposure to releases of naturally occurring substances and chemical additives. With the methodology and information currently available, it is also difficult to identify links between operational discharges and elevated levels of hazardous substances in organisms such as fish. Sandeels are a key species in the North Sea and are under growing pressure from existing and emerging ocean industries. Too little is known about the vulnerability of sandeels to various types of human-induced drivers, including pollution, and the possible impacts of these factors. Studies of the vulnerability of sandeels to crude oil are now being carried out by the Institute of Marine Research, financed by the petroleum industry.